Mini Review
For several decades we have been discussing possible harmful effects of manmade electromagnetic fields (EMFs) on human health, without being able to accurately highlight the existence of an intensity threshold (or frequency, or both) of EMFs not to be exceeded. However, it is a matter of fact that a huge number of studies on several effects on biological systems of EMFs emitted by human activities at very low intensities and regardless of the frequency of the field have been published so far. Furthermore, the fact that these effects have been significantly detected and quantified even on very simple organic systems such as proteins and cells leave no doubt as to the existence of such effects [1- 5]. Nevertheless, some researchers have pointed out that these effects do not find any theoretical explanation despite having been observed experimentally. In this regard, I would like to begin by citing the paper of Pierre and Dobson [6] in which it is expressly reported that the Lorentz force exerted on ions passing through cells membrane channels due to an applied EMF is negligible with respect to the forces that are needed to the activation of ions gating channel. In that paper the authors considered Na+ ion moving in cell channel at velocity v under a magnetic field (MF) B. Following Lorentz’s law, this charge should be subjected to the force F = qv× B .
The authors highlighted that a MF of 3 x 108 T is needed to induce a force of 1 pN and even considering ion velocity of 102 m s-1, a MF of 105 T would be necessary, which is several orders of magnitude larger than any magnetic field on Earth! Another study on the apparent contradiction between experimental observations of the effects of EMFs and negative theoretical results is that of Adair [7]. He made a simulation in which Hemoglobin (HB), chosen as protein prototype, is exposed to an EMF with amplitude E = 100 kV/m for Δt = 1 ns (the time integration from Sandia electric pulse, corresponding to the frequency of 900 MHz). The EMF will generate a torque on the protein because its significant dipole moment [8], inducing an angular impulse which has to compete with the mean angular momentum from thermal agitation to which the protein is subjected too. In this simulation, despite of the large value of amplitude of the EMF which was used, the mean angular momentum from thermal agitation resulted significantly larger than the impulse from EMF. Why do theoretical simulations contradict experimental observations? On the other hand, how is it possible that all the experimental results observed in recent years concerning the effects of EMFs are wrong? This apparent contradiction has been named the “kT problem”.
Let we consider macroparticles with a large dipole moment such as charged dust particles, mitochondria and some cells under exposure to an EMF. They will align towards the direction of the applied field: this phenomenon was named in literature “pearl chains” [9-13]. It can be easily explained by classical theory because the large mass of the organic particle gives rise to a large angular impulse that will overhead mean angular momentum from thermal agitation, as it can be easily shown. In contrast, kT problem arises when dealing with particles at the microscopic level such as molecules and ions. In this case, classical physics cannot longer be used, but a quantum approach should be used [14-23]. Indeed, light particles such electrons should have large wavelengths so that they can move from one side of a potential-energy barrier to the other side, despite of not having enough kinetic energy to overhead the barrier. However, in the second simulation above reported a macromolecule was taken in account so that even using classical physics a correct explanation should be found.
That is, it is a situation which is a cross between an atomic particle and a macroparticle, so that a semiclassical approach could apply. Instead, in the simulation reported above it seems that this cannot be done only because an adequate exposure time to EMF was not taken in account. For instance, in the first simulation reported above the integration time was not really considered and a constant intensity of MF was assumed. Nevertheless, even if the MF intensity has been assumed to be constant, the effects due to the applied MF should also depend on exposure time. Even if the torque induced by the applied MF is not intense enough to produce a displacement of the organic particle, however it will produce a very small displacement that will change the initial conditions from which the subsequent action of the force will begin in the following instants of time, In such a way that these imperceptible shifts will add up as the particle is exposed. An illustrative example of this situation can be represented by a (constant) force produced by the impact of a drop of water on a rock that does not produce any visible effect. However, the persistence of this action over time will produce considerable macroscopic effects on the rock that seem inexplicable by the single event, but which are instead produced by the sum of many events of this type. Regarding the second simulation above reported, instead, a time interval given by Sandia electric pulse Δt = 1 ns was taken in account, corresponding to the EMF frequency of 900 MHz. This choice depends on having considered a typical high frequency (HF) EMF. In this scenario the protein exposed to the EMF rotates from one side to the other following the frequency of the field, returning to the initial position after 1 ns, so that the average displacement is zero and no effect induced by the EMF could be explained.
However, this reasoning is correct if the particle rotates in a vacuum, whilst if it is embedded in a medium it cannot follow the EMF HF oscillation due to the inertia of particle and to the friction induced by the viscous damper of the medium in which the molecule is embedded. Hence, the particle exposed to the EMF will undergo a non-zero displacement correlated to the exposure time and a time interval larger than that of Sandia electric pulse should be taken in account. In the study published in [24] a macrodipole represented by the α-helix of typical proteins was considered to test this model, explaining the rotation of proteins α-helices and their alignment along the direction of the applied EMF observed by FTIR spectroscopy. This scenario can explain all the effects of exposure to EMFs of biological systems observed so far. Indeed, α- helix is present in all biological membrane channels and constitutes the walls of these channels [25-27], so that an applied EMF at very low intensity could not induce the rotation of these α-helices considering an integration time of the order of Sandia pulse, due to the significant binding forces in membrane channels.
In contrast, the impulse of the magnetic force due to an applied EMF calculated for the duration of exposure time can induce a torque on the α-helix causing a little increase in the diameter of cellular membrane channel [28-30]. This increase doesn’t have to be huge. A small variation is enough to produce an alteration in the flow of ions through cell membrane channel, causing alterations in cellular functions and the consequent effects that researchers have so far observed. Otherwise, it is true that the α-helix displacement must also compete with thermal molecular agitation, as Adair showed in his study [7]. However, even in this sense, considering an integration time comparable to a typical exposure time, it is easy to demonstrate that the magnetic torque exceeds the angular momentum from molecular thermal agitation [29,30]. A further study to explain experimental results of exposure to EMF by classical theory was carried out by the author and reported in [31], in which the competition between the mechanical moment due to a MF applied to HB protein and the diffusion quantity which is due to thermal molecular agitation was quantified. The complete equation of motion of a molecule subjected by both actions is given by

in which f(φ) is a distribution function and Φ is the rotational diffusion coefficient. The first two terms of this equation are due to the equation of diffusion process, the last term represents the quantity due to the mechanical moment and ω is the angular velocity of the molecule that can be calculated by the term
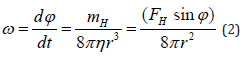
in which mH is the mechanical moment, FH is the force induced by the MF, r is the radius of the molecule and d = r sinφ is the distance between the application point of FH and the center of the molecule [32]. At the equilibrium, the torque of MF should balance thermal agitation so that we should have
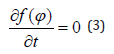
The solution of this equation is given by
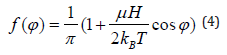
and the number of molecules that are rotated by an angle φ with respect to the direction of the field is given by

in which N is the total amount of paramagnetic molecules per unit of mass, μ is the molecular magnetic permeability and H is the applied MF [32].
The ratio of the numbers of molecules rotated with respect to the direction of the MF by two different angles φ1 and φ2 at the corresponding temperatures T1 and T2 is given by:

The parameters in the study of [31] were used to test the modeling above reported. As a result, the terms 2kBT1 and 2kBT2 can be neglected in comparison to μH and it can be assumed that cos φ1 = cos φ2 given that the displacement of molecules at the temperature T1 should be close to that at the temperature T2.
Finally, Eq. (6) can be written
so that the ratio between the number of molecules that are oriented along the direction of the applied MF by an angle φ is inversely proportional to the ratio between the temperatures. Such as showed in previous literature concerning FTIR spectroscopy, the intensity of the vibration band Amide I should be proportional to the number of proteins α-helices aligned with the direction of the applied MF. The ratios between the Amide I integrated area ratios of exposed/unexposed HB samples and some couple of temperatures T1 and T2 were computed in [31], providing the result that these values coincide with those obtained from Eq. (7), proving that the alignment of α-helix with the direction of an applied EMF is inversely proportional to the temperature. Above all, it was proved that the rotation of α-helix is not impeded by the Brownian motion due to thermal molecular agitation despite of the low intensity of MF used in [31], in agreement with the theoretical classical approach above reported.
Conclusion
Previous studies have highlighted that the effects caused by exposure of some organic systems to man-made electromagnetic fields at low intensities cannot be explained theoretically because angular momentum from molecular thermal agitation of surrounding medium is found to exceed the torque induced by EMF. Some authors assumed that a different approach by quantum physics is needed to solve this problem, in particular if we consider particles at atomic or subatomic size scales such as electrons or ions crossing cell membrane. However, if larger scales are considered, such as macromolecules like proteins, then classical physics can also be able to explain experimental results observed after exposure to man-made electromagnetic fields.
References
- Calabrò E, Condello S, Currò M, Ferlazzo N, Caccamo D, et al. (2013) Effects of Low Intensity Static Magnetic Field on FTIR spectra and ROS production in SH-SY5Y neuronal-like cells. Bioelectromagnetics 34(8): 618-629.
- Calabrò E, Magazù S (2015) Fourier –Self –Deconvolution Analysis of β-sheet Contents in the Amide I Region of Haemoglobin Aqueous Solutions under Exposure to 900 MHz Microwaves and bioprotective effectiveness of sugars and salt solutions. Spectroscopy Letters: An International Journal for Rapid Communication 48(10): 741-747.
- Calabrò E, Magazù S (2016) Parallel β-sheet Vibration Band Increases with Proteins Dipole Moment under Exposure to 1765 MHz Microwaves. Bioelectromagnetics 37(2): 99-107.
- Calabrò E (2016) Competition between Hydrogen Bonding and Protein Aggregation in Neuronal-Like Cells under Exposure to 50 Hz Magnetic Field. International Journal of Radiation Biology 92(7): 395-403.
- Calabrò E, Magazù S, Campo S (2012) Microwave-induced increase of amide I and amide II vibration bands and modulating functions of sodium-chloride, sucrose and trehalose aqueous solutions: The case study of Haemoglobin. Research Journal of Chemistry and Environment 16(4): 59-67.
- Pierre TGSt, Dobson J (2000) Theoretical evaluation of cell membrane ion channel activation by applied magnetic fields. Eur Biophys J 29(6): 455-456.
- Adair RK (2003) Biophysical limits on athermal effects of RF and microwave radiation. Bioelectromagnetics 24(1): 39-48.
- Antosiewicz J, Porschke D (1995) Electrostatics of hemoglobins from measurements of the electric dichroism and computer simulations. Biophys J 68(2): 655-664.
- Sher LD (1968) Dielectrophoresis in lossy dielectric media. Nature 220: 695-696.
- Schwan HP, Sher LD (1969) Alternating−current field−induced forces and their biological implications. J Electrochem Soc 116: 22C.
- Griffin JL, Ferris CD (1970) Pearl chain formation across radio frequency fields. Nature 226(5241): 152-154.
- Hu CJ, Barnes FS (1975) A simplified theory of pearl chain effects. Radiat Environ Biophys 12: 71-76.
- Takashima S, Schwan HP (1985) Alignment of microscopic particles in electric fields and its biological implications. Biophys J 47(4): 513-518.
- Lednev VV (1991) Possible mechanism for the influence of weak magnetic fields on biological systems. Bioelectromagnetics 12(2): 71-75.
- Panagopoulos DJ, Messini N, Karabarbounis A, Philippetis AL, Margaritis LH (2000) A mechanism for action of oscillating electric fields on cells. Biochemical and Biophysical Research Communications 272(3): 634-640.
- Binhi VN, Savin AV (2003) Effects of weak magnetic fields on biological systems: physical aspects. Physics-Uspekhi 46(3): 259-291
- Binhi VN, Rubin AB (2007) Magnetobiology: the kT paradox and possible solutions. Electromagn Biol Med 26(1): 45-62.
- Halgamuge MN, Perssont BRR, Salford LG, Mendis P, Eberhardt J (2009) Comparison between two models for interactions between electric and magnetic fields and proteins in cell membranes. Environmental Engineering Science 23(10): 1473-1480.
- Lauer V (2013) A Quantum Theory of the Biological Effects of Radio-frequencies and its application to Cancer. hal-00877298v2
- Drozdov AV, Nagorskaya TP, Masyukevich SV, Gorshkov ES (2010) Quantum mechanical aspects of the effects of weak magnetic fields on biological objects. Biophysics 55(4): 652-660
- Fleming GR, Scholes GD, Cheng YC (2011) Quantum effects in biology. Procedia Chemistry 3: 38-57.
- Karimpour MR, Fedorov DV, Tkatchenko A (2022) Molecular Interactions Induced by a Static Electric Field in Quantum Mechanics and Quantum Electrodynamics. J Phys Chem Lett 13(9): 2197-2204.
- Hadi Zadeh Haghighi H, Simon C (2022) Magnetic field effects in biology from the perspective of the radical pair mechanism. J R Soc Interface 19(193): 20220325.
- Calabrò E, Magazù S (2017) The α-Helix Alignment of Proteins in Water Solution towards a High Frequency Electromagnetic Field: a FTIR Spectroscopy Study, Electromagnetic Biology and Medicine 36(3): 279-288.
- Becker L, Bannwarth M, Meisinger C, Kirstin Model, Thomas Krimmer, et al. (2005) Preprotein translocase of the outer mitochondrial membrane: Reconstituted Tom 40 forms a characteristic TOM pore. J Mol Biol 353(5): 1011-1020.
- Feria Bourrellier AB, Valot B, Guillot A, Jean Vidal, Michael Hodges, et al (2010) Chloroplast acetyl-CoA carboxylase activity is 2-oxoglutarate-regulated by interaction of PII with the biotin carboxyl carrier subunit. Proc Natl Acad Sci USA 107(1): 502-507.
- Szabo I, Zoratti M (2014) Mitochondrial channels: Ion fluxes and more. Physiol Rev 94(2): 519-608.
- Calabrò E, Magazù S (2018) Resonant interaction between electromagnetic fields and proteins: A possible starting point for the treatment of cancer. Electromagnetic Biology and Medicine 37(3): 155-168.
- Calabrò E, Magazù S (2019) Infrared Spectroscopic Demonstration of Magnetic Orientation in SH-SY5Y Neuronal-Like Cells Induced by Static or 50 Hz Magnetic Fields. International Journal of Radiation Biology 95(6): 781-787.
- Calabrò E, Magazù S, Currò M, Ientile R (2020) The inverse relation between mitochondrial transmembrane potential and proteins α-helix in neuronal-like cells under static magnetic field and the role of VDAC. Electromagnetic Biology and Medicine 39(2): 176-182.
- Calabrò E, Magazù S (2018) Direct spectroscopic evidence for competition between thermal molecular agitation and magnetic field in a tetrameric protein in aqueous solution. Physics Letters A 382(21): 1389-1394.
- Valentinuzzi M (1996) Rotational Diffusion in a Magnetic Field and its Possible Magnetobiological Implications. Chapter 5 in “Biological Effects of Magnetic Fields”, In: MF Barnothy (Edt.)., Springer, New York.