ABSTRACT
The toxicological effects of prepared neem oil nano-formulations compared with the bulk one on Swiss male albino mice were investigated. Characterization of the prepared nano-formulations using Transmission Electron Microscopy (TEM) revealed that the size of the prepared nanoparticles ranged between 30-50 and 60- 80 nm for nano-emulsion and loaded nano-emulsion particles, respectively. The acute oral LD50 values were 113.33, 134.83, and 140.90 mg/kg for loaded nano-emulsion, nano-emulsion, and bulk neem extract, respectively. The oral administration of the sub-lethal dose (LD10) caused significant alterations in the selected biomarkers. Hemoglobin percent and Red Blood corpuscles (RBCs) count decreased significantly after the seventh day and White Blood Cells (WBCs) count reduced after the third day of treatment by loaded nano-emulsion treatment. Aspartate aminotransferase and bilirubin increased significantly after the seventh day in nano-emulsion and loaded nano-emulsion treatment, respectively. Alanine aminotransferase significantly decreased after the seventh day of treatment by nano-emulsion. Compared to the control values, glutathione S-transferase decreased to -52.21 %, while reduced glutathione increased to +42.18 % after the seventh day in nano-emulsion treatment. Serum creatinine increased to +135.71 % after the seventh day in loaded nanoemulsion and total ATPase increased to +8.56 % after the third day in nano-emulsion treatment.
Keywords: Toxicity; Neem Nano-Formulations; Swiss Male Albino Mice; Biomarkers
Introduction
A little information is available concerning the safety and/or the adverse effects of the prepared nanoparticles on the mammalian organisms and hence the application of nanotechnologies in the agro-food sector (Buzea, et al. [1,2]). Several researchers have used nanotechnology to modify the composition, action, and delivery of formulations of different products of natural (botanical) origins which affect and/or improve in turn the efficiency of the final product against the targeted pests. Many botanicals including natural protectants have not been thoroughly investigated for their mammalian toxicity. Some of the plants derived insecticides such as azadirachtin, nicotine, pyrethrin, rotenone, ryania, sabadilla, and spinosad have been evaluated for mammalian safety (Rajashekar, et al. [3]). The extract of neem tree Azadirachta indica, contains phenol, the antioxidant effects of phenolic compounds are related to the number of different mechanisms, such as free radical-scavenging, singlet oxygen quenching, metal ion chelation, hydrogen-donation and their action as substrates for free radicals such as superoxide anion and hydroxyl radical which may affect their toxic action toward the non-targeted organisms (Sharma, et al. [4,5]). At this moment there are too little data to determine which type of effects are to be expected for which type of nanoparticles (NPs) (Balbus, et al. [6,7]).
It is well known that there is a lack and/or shortage of the toxicological information on different prepared nanoparticles. In such conception, studies of the toxicity of nano-particles as pointed out by Elsaesser and Howard [8] showed that there was a need for further research concerning the synthesis of new materials and evaluation of their toxicity. New combinations of materials may exhibit toxic effects different from those observed in earlier studies (Shinde et al. [9]). In addition, it was found that physicochemical properties may be important in understanding the toxic effects of tested materials, i.e. particle size and size distribution, agglomeration state, shape, crystal structure, chemical composition, surface area, surface chemistry, surface charge and porosity (Oberdorster, et al. [10,11]). Also, it was proved that increased surface reactivity predicts that NPs exhibit greater biological activity per given mass compared with larger particles which they should be taken up into living organisms. This increased biological activity can be either positive or desirable (e.g., antioxidant activity, carrier capacity for therapeutics, penetration of cellular barriers for drug delivery) or negative and undesirable (e.g., toxicity, induction of oxidative stress or cellular dysfunction), or a mix of both (Oberdorster, et al. [12]). From another viewpoint, it is well known that neem extract derived from the tree (Azadirachta indica) is the most active plant extract among others. Its insecticidal activity is based on its mode of action as interfering with larval growth and development, growth retardation, molting inhibition, or induction of malformation in the larval stage. In the adult insect, such extracts inhibit egg maturation and may also induce sterilization in several insect species and such effects can also be induced as hormone application (Dimetry, et al. [13,14]).
To overcome the disadvantages of the traditional botanical extract, i.e. instability, chemical decomposition, and hence loss of its insecticidal properties, green nano-technology was believed that such technology may be a successful way which is recently used to improve the pesticidal properties of a botanical insecticide. Nanotechnology has grown to be an important research field in all areas. The size, orientation, and physical properties of nanoparticles have reportedly been shown to change the performance of any material (Mubayi, et al. [15]). In case of the toxic effect of the neem extract on a mammalian model, there are contradictions between the published reports. For example, it was found that acute oral toxicity of ethanol neem extract containing 3000 ppm azadirachtin (±10 %) is registered with the Environmental Protection Agency (EPA), USA and the data submitted on acute oral toxicity in rats showed no negative effect up to a dose of 5 mL/kg as described by National Academy Press, Washington D.C. (Anonymous [16]). In another study, methanolic leaf and bark extracts showed an oral LD50 of about 13 g/kg in acute toxicity studies on mice. Also, (Raizada, et al. [17]) administrated a single oral dose of azadirachtin (5000 mg/kg) to male and female rats produced neither any signs of toxicity nor death in the animals. The LD50 value, therefore, is more than 5000 mg/kg both in male and female rats. For Neemazal (Neem-based pesticidal product) LD50 values were higher than 2g/kg BW in mice (Niemann, et al. [18]). However, acute, subacute, and subchronic toxicity following oral exposure has been investigated in rodents for several NPs. (Wang, et al. [19,20]) Stated that the available oral toxicity studies indicated that, depending on the particle size, coating and chemical composition of the NPs, acute toxicity at high doses may occur.
Conversely, in case of the chronic toxicity of neem extract, most of the neem-based products are toxic. For Praneem and Nimbokil-60 effects on reproduction and fertility were reported. All other agents administered once or chronically, negatively influence animal health and in some cases even cause death with medium lethal concentration (LC50) values varying in the range from 1.6 to 16 mg/kg (Bray, et al. [21]). Based on the above-mentioned facts, the present study aimed to study the toxic effects of three different formulations, bulk, nano-emulsion, and loaded nano-emulsion of neem extract to investigate the adverse effects of the prepared neem nano-formulation on different biomarkers, i.e. RBC’s, WBC’s, hemoglobin (as hematological parameters), Aspartate Aminotransferase (AST), Alanine Aminotransferase (ALT), bilirubin content, Glutathione S-transferase (GST), reduced glutathione (GSH), serum creatinine and total Adenosine Triphosphatase (ATPase) activity using Swiss albino male mice as a mammalian model.
Materials and Methods
Chemicals
All chemicals used in this work i.e. tween 80, sodium alginate, methanol which is used in the preparation of nano-formulations, diethyl ether used in anesthesia of treated mice, and all chemicals used in some enzymatic activity evaluation, total ATPase and glutathione S-transferase, glutathione and protein content, i.e. Coomassie Brilliant blue G-250, ethanol, phosphoric acid, bovine serum albumin, sodium dihydrogen phosphate disodium hydrogen phosphate, glutathione, 1-chloro-2, 4-dinitro benzene (CDNB), [(5, 5-dithiobis (2-nitrobenzoic acid)] (DTNB), trichloroacetic acid, Tris–Mes, ethylene glycol bis (β-aminoethyl ether), N, N-tetra acetic acid (EGTA), dithiothreitol, potassium chloride, sodium azide, sodium dodecyl sulfate (SDS), sulphuric acid, ammonium molybdate, antimony potassium tartrate, and ascorbic acid were purchased from Sigma Chemical Co. (Missouri, USA). The other enzymatic activities, i.e. Aspartate aminotransferase (AST), Alanine aminotransferase (ALT), and bilirubin content were evaluated using a kit purchased from Biogiagnostic Company, (Giza, Egypt). Creatinine content was determined using kits purchased from Salucea company, (Giza, Egypt).
Preparation of Nano-Formulations
Nano-Emulsion Preparation: Neem oil 0.03 % azadirachtin obtained from (Department of Pests and Plant Protection, National Research Centre) was used in this investigation. Neem oil, Tween 80 and distilled water were used in the preparation of emulsion by a modification of the method described by (Jerobin, et al. [22]). Nano-emulsion preparations were prepared by diluting the neem oil with distilled water in a ratio of 1:1 (oil: water). Two percent of Tween 80 was added as an emulsifier. The formed emulsion was sonicated for 30 min using an ultrasonic cleaner set, (model WUCDO3H 290W and 60 Hz, Wertheim, Germany), then sonicated for 1 min using a high energy ultrasonication probe, (model VCX750, 750W, 20 kHz, Newtown, USA), and re-sonicated for 30 min by the ultrasonic cleaner under cooling conditions using ice path. In another experiment, neem oil was sonicated using the same abovementioned ultrasonic cleaner set only for I hour without using a high energy ultrasonication probe.
Preparation of Loaded Nano-Emulsions: Alginate nanocapsules were prepared using oil in water (o/w) emulsification, followed by crosslinking using calcium chloride and solvent removal (in the case of using solvent), using modified versions of the methods described by (Bouchemal, et al. [23]) and (Lertsutthiwong, et al. [24]). Sodium alginate solution (3 %, w/v) was prepared by dissolution in distilled water at 50˚C for 45 min. The tested oil was diluted by distilled water using Tween 80 as an emulsifier with mechanical stirring for 10 min. Briefly, the sodium alginate o/w emulsion was made by dropwise dispersion of diluted oil into an appropriate volume of alginate solution (1:1 oil to alginate) under continuous mechanical stirring at room temperature. The emulsion thus formed was sonicated for 30 min using the same above-mentioned ultrasonic cleaner set and then sonicated for 2 min using the same mentioned high energy ultrasonication probe. An appropriate volume of CaCl2 (2:10 CaCl2 to alginate, respectively) was then added to the resulting emulsion and stirred for an additional 30 min and sonicated as mentioned previously. The loaded nanocapsule suspension was equilibrated overnight. Nano-capsules were obtained as a dispersion in an aqueous solution.
Characterization of Nano-Formulations: To ensure that the prepared neem oil formulations have become in the nano-sized particles, different methods of characterization were followed up to measure the morphological shape, size, uniformity content, and chemical interactions of the obtained nano-formulations and/or nanoparticles.
Transmission Electron Microscopy (TEM): The morphological shapes of prepared nano-formulations were tested using Transmission Electron Microscopy (TEM) (Jeol, JEM-210, Tokyo, Japan). The nano-capsule suspensions were diluted with distilled water and deposited onto a carbon-coated copper grid and stained with a 1 % phosphotungstic acid then examined by magnification (20000X) and photographed (Rivera, et al. [25]) (Figure 1).
Figure 1: Transmission electron microscopy (TEM) images,
a) Neem nano-emulsion and
b) Neem loaded nano-emulsion.
UV Spectrophotometric Examinations: Loaded nano-capsules were evaluated for the active ingredient contents (azadirachtin in neem oil) and this was carried out by refluxing 0.75 gm of the loaded nano-emulsions with 10 mL of methanol at 65 OC. Refluxing was continued for 1 h, to ensure complete extraction of encapsulated oil. The samples were then centrifuged at 10.000 xg for 10 min. The absorbance of methanol containing the extracted amount of azadirachtin was taken at a wavelength of 214 nm (Jerobin, et al. [22]) and extracted the amount of menthol was taken at a wavelength of 230 nm (Xue, et al. [26]) in a UV spectrophotometer, (CHEM-7, California, USA), using absolute methanol as a blank. Oil concentration was calculated with the use of a calibration curve obtained from samples of pure oil within a certain concentration range of neem oil. The encapsulation parameters were determined according to (Kumar, et al. [27]) as follows:
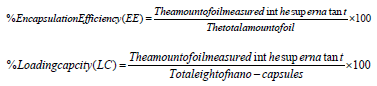
Fourier Transforms Infrared (FTIR) measurements: FTIR measurements were carried out to detect any chemical interactions between neem oil nano-emulsion and Na-Alg or the cross-linking agent CaCl2. This was carried out by taking the following samples from both tested Neem oil: pure bulk oil, oil nano-emulsion, and loaded Nano-emulsion. The FTIR measurements were carried out using FTIR 6600 (JASCO, Tokyo, Japan) according to the method described by (Jerobin, et al. [22]).
Toxicological Effects of Neem Oil Formulations on Swiss Male Albino Mice: To investigate the toxic effect of the prepared neem formulation on the mammalian organisms, inbred normal Swiss albino male mice weighing 20-25 g were used throughout this study. Mice were obtained from the central animal house of the National Research Centre. The mice were kept in the Departmental Animal House at 26±2 °C and relative humidity of 44-56 %, with light and dark cycles of 10 and 14 hr respectively. Animals were provided with a standard rodent pellet diet. Food and water were allowed ad libitum.
Acute Oral LD50 Toxicity Test: An acute oral toxicity study for calculating LD50 was carried out. Animals have been divided into four groups each of three mice. The tested groups were orally treated using a gavage needle with neem formulations at the doses of 81.93, 106.51, 138.46, and 180.00 mg azadirachtin (aza)/kg body weight (BW). The control group was treated with distilled water. Following oral administration, the animals were observed for mortality during the first 24 h. The lethal dose (LD50) was estimated according to the method described by (Weil [28]).
Sub-Acute Toxicity Study: Doses of LD10 were calculated for each neem formulation, i.e. bulk, nano, and loaded nano-emulsion to be used in the sub-lethal tests. Three groups of 18 male mice received a daily dose of 28.18 mg aza/kg BW for bulk neem oil, 26.97 mg aza/kg BW for neem oil nano-emulsion, and 22.67 mg aza/kg BW for loaded nano-emulsion for seven days. After the seventh day, recovery periods of two weeks were estimated without any treatments. The oral administration time course of the tested formulations was followed according to the method described by (Hayes [29]).
Determination of Hematological and Clinical Biomarkers: During the experimental period, samples of blood, serum, liver, and brain were collected after anesthesia using diethyl ether and dissection of the treated mice on the first, third, fifth, seventh, fourteenth, and twentieth day. Tissue samples have been weighed and homogenized in 0.1 M phosphate buffer (pH 7.0) a ratio of 1:2 w/v. The resultant homogenate was centrifuged at 10.000 xg /4˚C/ 30min. The resultant supernatant was obtained and stored at -80˚C until assaying. Red Blood Corpuscles (RBCs), White Blood Cells (WBCs), and hemoglobin were determined in fresh blood samples according to the methods of (Drabkin, et al. [30]). As hepatotoxicity biomarkers, Aspartate Aminotransferase (AST) and Alanine Aminotransferase (ALT) were determined according to the method of (Reitman, et al. [31]). Glutathione S-transferase (GST) was determined spectrophotometrically according to the method of (Habig, et al. [32]) while reduced glutathione (GSH) content in the liver was measured according to the colorimetric method of (Ellman [33]). Bilirubin content was determined in serum samples according to (Walter, et al. [34]). As a nephrotoxicity biomarker, serum creatinine was evaluated according to the method of (Tobias, et al. [35]). In addition, the activity of total Adenosine Triphosphatase (ATPase) activity was measured according to the method of (Sarkadi, et al. [36]). Total protein determination was carried out according to the method described by (Bradford [37]).
Statistical Analysis: Data were subjected to statistical analysis by one-way analysis of variance (ANOVA) using SPSS software (Tukey test). A value of p < 0.05 was considered statistically significant.
Results
Characterization of Nano-Preparations
Electron Microscopy Examination: The prepared neem nanoformulations, i.e. nano-emulsions and loaded nano-emulsions, were examined using a Transmission Electron Microscope (TEM). Data from TEM showed that nanoparticles diameters ranged between 30-50 nm and almost all the prepared nanoparticles were spherical with a smooth surface (Figure 1a). In the case of the prepared alginate nanocapsules containing neem oil (loaded nano-emulsion), the particle diameters were bigger than those of emulsion particles and increased to be 60-80 nm (Figure 1b).
Fourier Transforms Infrared (FTIR) Measurements: The chemical interactions among the ingredients i.e., sodium alginate (Na-Alg) and botanical oils nano-emulsions were studied to understand the stability of nano-emulsion inside the matrix system. FTIR data is represented in (Figure 2) for neem oil (curve a); neem oil nano-emulsion (curve b) and neem oil loaded nanoemulsion (curve c) and summarized in Table 1. The characteristic sharp peaks of neem oil were at 3455.81 (O-H), 2925.48 (aromatic and/ or vinylic C-H), 2854 (aliphatic C-H), and 1037 (various C-O), respectively.
Figure 2: FTIR spectra of
a) Pure neem oil,
b) Neem oil nano-emulsion and
c) Neem oil loaded alginate nano-emulsion.
Table 1: Summarizing of major chemical groups FTIR spectra of the neem oil bulk and nano-formulations.
Determination of Encapsulation Efficiency (EE) and Loading Capacity (LC) of prepared loaded nano-formulations: Results of incorporation of neem oil into alginate nano-capsules to prevent the rapid evaporation, and degradation and enhance their stability showed that the encapsulation efficiency (%EE) increased with the loaded emulsion prepared with distilled water than those prepared using ethanol. The EE was 75.53% and 21.49%, respectively. On the other hand, loading capacity (%LC) showed the same significant differences between the two treatments, they were 1.99% and 1.40%, respectively.
Toxicity of the Prepared Neem Nano-Formulations on Swiss Male Albino Mice: The toxicological effects of the prepared neem nano-formulations compared with the bulk one on swiss male albino mice were investigated through the determination of acute oral LD50 and study of the toxic effect of sublethal dose (LD10) on some biochemical parameters.
Table 2: LD50 and LD10 values of the prepared neem nanoemulsion, loaded nano-emulsion and bulk formulations, determined after 24 hr of oral administration to Swiss male albino mice.
Acute Oral Dose LD50: The acute oral LD50 and sub-lethal LD10 values of bulk, nano-emulsion, and loaded nano-emulsion were determined using Swiss male albino mice. Data listed in Table 2 showed the determined LD50 values which reached 113.33, 134.83, and 140.90 mg/kg body weight for loaded nano-emulsion, nanoemulsion, and bulk formulations, respectively. Such values indicate that the loaded nano-emulsion was the most toxic formulation than the other two tested formulations. The same trend was observed when the sublethal dose (LD10) values were determined. Such doses were calculated at 22.67, 26.97, and 28.18 mg/kg for the loaded nano-emulsion, nano-emulsion, and the bulk formulation, respectively. Thus, the loaded nano-emulsion exhibited the most toxic prepared nano-formulation of the oil neem extract.
Toxicity of Sub-Lethal Dose (LD10) of the Tested Neem Bulk and Two Nano-Formulations on Some Biomarkers: To investigate the toxic effects of the prepared bulk and two nano-formulations of neem extract, the tested formulations were administrated to Swiss male albino mice orally at their corresponding sublethal dose (LD10) for seven days (subacute administration) and the exposure procedure was stopped during two weeks (as a recovery period) based on the guideline described by (Hayes [29]). To explore the toxic effects of the tested formulations, some biochemical markers were targeted and measured during the subacute treatment, i.e. hematological, hepatotoxicity, and renal biomarkers.
Hematological Biomarkers: The effect of the tested neem formulations was examined on different hematological biomarkers, i.e. Hemoglobin, Red blood corpuscles (RBCs), and White blood cells (WBCs). Data in Table 3 showed that hemoglobin levels were altered significantly in some treatments while not affected in other ones. For example, after treatment of the albino mice with the bulk formulation, the hemoglobin increased significantly, after the first and third days of continuous treatment to reach +39.61 and +107.53 % of control values, respectively, while on the twentieth day (after 14 days of recovery period) it was elevated to be +52.84 % of control value. When the albino mice were treated with the neem nano-emulsion, a significant increase was recorded after three days of continuous treatment to be +99.73 % of control values and after two weeks of ending the treatment (the twentieth day) to be +20.31 of the control value. In the case of loaded nano-emulsion, the hemoglobin level was affected significantly to be evaluated at +77.81 % of the control value on the third day of continuous exposure. On the seventh day, the hemoglobin level was decreased significantly to be -55.95 % of the control value, while after one and two weeks of ending the treatment (the fourteenth and twentieth day), the hemoglobin level was returned to be increased significantly to be +32.63 and +86.24 %, respectively, of the control value. However, the hemoglobin levels were not significantly affected in the other treatments.
Table 3: Levels of hemoglobin, RBCs and WBCs, during the sub-acute oral administration of the sub-lethal doses (LD10) of the tested neem nano-formulations on Swiss male albino mice.
Note: *Each value of the biomarker level represents the mean of 3 replicates ± S.E. Values between brackets represent the percentage of increase or decrease compared to the control animals. Values with different letters within the same Column are significantly different (p. ˂ 0.05) (Tukey test).
In the case of Red Blood Corpuscles (RBCs), such parameter was affected significantly in all treatments. For example, their counts were significantly increased after treating the mice with the bulk formulation to be +103.41 % and +6.12 % of control values after the first and third day of the continuous exposure to the corresponding LD10 value, respectively, but returned to decrease to be -14.48 %, in comparison with the control after the fifth day of continuous treatment. When the male albino mice were exposed to the nano-emulsion, data listed in Table 3 indicated that the count of (RBCs) was decreased significantly after the first, fifth, and seventh day of the continuous treatment by the corresponding LD10 value to be -20.04 %, -60.49 % and -19.50 % related to control value, respectively. While on the third day it was increased to be +42.25 %. After one week of the recovery period, the (RBCs) turned back to increase significantly to be +80.00 % of the control value. Treatment by loaded nano-emulsion, the same trend was observed, i.e. significantly increased after the first and third day to be +67.80 % and +343.89 % in (RBCs) counts to control value. While, there was decreasing to be -29.63 % and -83.50 % of the control value after the fifth and seventh day of continuous exposure, respectively. Also, on the the fourteenth and twentieth days (one and two weeks of recovery period) the count of RBCs increased significantly to be +64.63 % and +10.18 %, respectively Table 3.
White Blood Cells (WBCs) were counted in the blood samples collected after exposure of Swiss albino mice to the tested formulations, some significant alterations were recorded. For example, bulk formulation caused a significant decrease in the (WBC) counts to reach -51.45 %, -49.85 %, -78.57 %, and -44.72 % of control value after the first and third day of continuous exposure, fourteenth and twentieth, respectively. On the contrary, WBC counts were elevated to reach +150.00 % of the control value after the fifth day of continuous exposing the albino mice to the nanoemulsion. Such formulation caused a significant decrease in the counts of (WBCs), to be -75.82 %, -30.03 %, -50.00 %, and -72.46 % of control value after the first and third day of continuous exposure, day fourteenth and twentieth day of a recovery period, respectively. The only insignificant increase in such parameter reached +50.00 % after the fifth day of continuous exposure. In addition, the loaded nano-emulsion caused a significant decrease in the counts of WBC reaching -69.64 %, -60.06 %, and -48.24 % of control value after the first and third day of continuous exposure and twentieth day (two weeks) as a recovery period, respectively.
Hepatotoxicity Biomarkers: The effect of the three tested neem formulations, i.e. bulk, nano-emulsion, and loaded nano-emulsion were studied on various hepatotoxicity biomarkers which included, Aspartate aminotransferase (AST), Alanine aminotransferase (ALT) which used as biomarkers for liver function and bilirubin Table 4 while glutathione S- transferase and reduced glutathione Table 5.
Table 4: The Specific activities of AST, ALT and bilirubin content and their corresponding activity and/or level percentage during the sub-acute oral administration of the sub-lethal doses (LD10) of the tested neem nano-formulations on Swiss male albino mice.
Note: *Each value of the biomarker level represents the mean of 3 replicates ± S.E. Values between brackets represent the percentage of increase or decrease compared to the control animals. Values with different letters within the same Column are significantly different (p. ˂ 0.05) (Tukey test).
Table 5: The Specific activities of glutathione S-transferase (GST) and content of reduced glutathione (GSH) during the sub-acute oral administration of the sub-lethal doses (LD10) of the tested neem nano-formulations on Swiss male albino mice.
Note: *Each value of the biomarker level represents the mean of 3 replicates ± S.E. Values between brackets represent the percentage of increase or decrease compared to the control animals. Values with different letters within the same column are significantly different (p. ˂ 0.05) (Tukey test).
Serum Aspartate Aminotransferase (AST) and Alanine Aminotransferase (ALT): Data listed in Table 4 showed that the bulk formulation of neem caused a significant decrease in (AST) activity to reach -2.80 % and returned to a significant increase to reach +57.27 %, +8.98 %, and +3.02 % of the control value after the first, third, seventh day (of continuous exposure) and at the twentieth day (two weeks of recovery period), respectively. Nanoemulsion formulation caused a significant elevation in the (AST) activity reaching 5.13 % and 15.63 % after the fifth and seventh day of continuous exposure, respectively. Also, there was an increase in the AST activity represented by 12.32 % after one week of the recovery period. A Significant decrease determined by -5.41 % of the control value on the twentieth day (two weeks of recovery period) was observed. When the Swiss albino mice were tested with the loaded nano-emulsion, it was found that such formulation caused a significant decrease in (AST) activity reached to -6.26 %, -5.95 % while a significant increase was recorded reached to +8.96 %, +14.41 %, and 9.33 % of control value after first, the fifth and seventh day of continuous treatment and fourteenth and twentieth day (one and two weeks) of the recovery period, respectively.
In the case of (ALT) activity, such parameter was affected significantly only after the third and seventh day of continuous exposure to the bulk formulation at the fourteenth day (one week of recovery period) to reach an increase determined by +23.98 % and decrease reached to -44.74 % and -47.20 % of the control value, respectively. The nano-emulsion caused a significant decrease in (ALT) activity reached to -47.45 % and -45.01 % on the seventh day of treatment and fourteenth day (one week of recovery period), respectively. In addition, the loaded nano-emulsion caused a significant increase reached to +20.04 % on the third day of treatment, while causing a significant decrease reached to -45.35 % and -51.56 % of the control value on the seventh day of treatment and fourteenth day (one week of recovery period), respectively.
Serum Bilirubin: Data listed in Table 4 indicated that levels of bilirubin were significantly affected in some treatments after exposure of the albino mice to the tested formulations. For example, neem bulk formulation caused alteration varied between increasing and decreasing in the bilirubin levels. Such levels reached +50.48 %, -53.70 %, +78.38 %, and +355.88 % of control value after the first, third, and fifth day of continuous exposure and on the twentieth day (two weeks of recovery period), respectively. Nano-emulsion caused a significant increase only on the fifth day of continuous treatment and the twentieth day determined by +135.14 % and +138.24 % of the control value, respectively. In the same manner loaded nano-emulsion caused a significant elevation in bilirubin levels to reach +101.90 %, +81.82 %, +330.19 %, and +144.12 % of control value at the first and seventh day of continuous exposure, fourteenth and twentieth day (one and two weeks of recovery period), respectively.
Hepatic Glutathione S-Transferase (GST) and Reduced Glutathione (GHT): Data in Table 5 showed the specific activity of glutathione S-transferase in the liver expressed as (nmol/min/ mg protein) after exposing the albino mice to the three tested neem nano-formulations. The subacute oral administration of such formulations caused a significant alteration in the activity of such enzymes varied between elevation and decrease. In the case of neem bulk formulation caused a decrease (-53.10 %), elevation (+241.38 %), (+86.73 %), decline (-73.61 %), and (-92.01) at the first, fifth and seventh day of continuous exposure and after the fourteenth and twentieth day, respectively. Nano-emulsion formulation caused a converse trend, i.e. increase in (GST) activity on the first day (+59.29 %), a decrease on the fifth day (-27.59 %), and the seventh day (-52.21 %) of continuous treatment, and a significant decrease determined by (-81.94 %) and (-93.06 %) of control value after the fourteenth and twentieth day, respectively. A similar trend to bulk formulation was observed by loaded nanoemulsion which caused a significant decrease in (GST) activity determined by -82.30 % on the first day which turned to be elevation calculated by +237.93 % and +163.72 % of control value at the fifth and seventh day of continuous treatment and turned to decrease significantly to be -54.17 % and -71.18 % of control value at the fourteenth and twentieth day, respectively. In the case of liver reduced glutathione (GSH), it is clear that the levels of such parameters were affected significantly but not at all during the experimental periods, as a result of sublethal exposure. Neem bulk formulation caused a significant elevation in (GSH) level on day 3 of continuous treatment (+33.24 %) and such level was decreased on the fourteenth day (after one week of recovery period) (-22.64 %) of the control value. Neem nano-emulsion caused a significant decrease and then elevation determined by -42.97 %, +86.85 %, +42.18 % of control value at the first, fifth, and seventh day of continuous treatment, respectively. In addition, neem-loaded nanoemulsion caused a significant decrease (-55.94 %, increase (+44.68 %) and decrease (-22.31 %) of control value at the first, fifth, and seventh-day continuous exposure, respectively.
Serum Creatinine: Data related to the levels of serum creatinine as a biomarker of renal function are listed in Table 6. They indicated that the levels of such parameters were dramatically altered between significant increase and decrease. For example, the neem bulk formulation caused significant elevation determined by +128.57 %, +59.82 %, and +121.30 % of control value on the third and seventh day of control treatment and the twentieth day (two weeks of recovery period), while the same formulation caused significant decrease calculated by -52.68 % and -45.66 % of control value on the fifth day of continuous treatment and the fourteenth day (one week of recovery period), respectively. Almost the same trend of results was obtained after treatment with the nanoemulsion which caused a significant increase in serum creatinine reached to +114.29 % and +237.04 % of control value on the third day of continuous treatment and the twentieth day (two weeks of recovery period), respectively. Conversely, the same formulation caused a significant decrease on the seventh day of continuous treatment and the fourteenth day determined by -76.79 % and -17.81 % of the control values, respectively.
Table 6: Creatinine content and its corresponding percentage during the sub-acute oral administration of the sub-lethal doses (LD10) of the tested neem nano-formulations on Swiss male albino mice.
Note: *Each value of the biomarker level represents the mean of 3 replicates ± S.E. Values between brackets represent the percentage of increase or decrease compared to the control animals. Values with different letters within the same Column are significantly different (p. ˂ 0.05) (Tukey test).
In The case of subacute administration of neem-loaded nanoemulsion, such formulation caused also dramatic alterations in creatinine levels. In terms of figures, serum creatinine decreased significantly to -34.57 %, -60.71 %, and -26.48 % of control value on the first and fifth day of continuous treatment and the fourteenth day (one week of recovery period), respectively. Conversely, the level of the parameter was significantly increased by +100.00%, +135.71 %, and +330.56 % of control value on the third and seventh day of continuous exposure and the twentieth day (two weeks of recovery period), respectively.
Total ATPase: The effect of the tested neem nano-formulations was examined on ATPase-specific activity. Data in Table 7 showed that the activity of total ATPase was altered significantly in some treatments while not affected in other ones. For example, after treatment of the albino mice with bulk formulation, the ATPase specific activity reduced significantly to be -6.68 % after one day, then it was increased to be +5.14 % after the third day, while it was not affected significantly after the fifth, seventh, fourteenth and twentieth day as it was -2.35 %, +0.12 %, +2.92 % and +0.08 % of the control value, respectively. When the albino mice were treated with neem nano-emulsion, the ATPase activity was affected significantly after days 1 and 3 which reached -6.31 % and +8.56 %, respectively. After the fifth, seventh, fourteenth, and twentieth day, the specific activities were not affected significantly and were -5.46 %, +2.81 %, +4.32 %, and +1.63 %, respectively from the control values. In the case of loaded nano-emulsion, the specific activities were affected after the first and third days which reached +3.66 and +7.12 %. After the fifth, seventh, fourteenth, and twentieth day the specific activities were not affected significantly and were -1.45 %, +0.98 %, +2.40 %, and +3.03 %, respectively.
Table 7: The specific activity of total ATPase and its corresponding activity percentage during the sub-acute oral administration of the sub-lethal doses (LD10) of the three tested neem nano-formulations in Swiss male albino mice.
Note: *Each value of the specific activity represents the mean of 3 replicates ± S.E. Values between brackets represent the percentage of increase or decrease compared to the control larvae. Values with different letters within the same column are significantly different (p. <0.05) (Tukey test).
Discussion
Based on the fact that the toxic effects of whatever nanoparticles are related to the characteristics of the tested particles, so, the characterization procedure could be considered a key factor to investigate the tested nanoparticles either synthesized or of natural origin. According to the obtained results related to the characterization process of the prepared nanoparticles, using TEM, the size of the prepared nanoparticle of the tested neem oil, the resultant sizes may be due to the sonication time and energy used during such procedure. Several authors suggested that increasing sonication time and energy were the main reason for decreasing the size of nanoparticles. For example, (Ghotbi, et al. [38]) found that droplet size decreased with the increase in surfactant concentration and time of sonication when they prepared waterbased nano-emulsions from neem seed oil using Tween 20 as a surfactant. Similar results were obtained by (Kale, et al. [39,40]). As for the other characterization processes, the results of FTIR measurements indicated that there is no chemical interaction, a similar finding was reported by (Kulkarni, et al. [41,42,22]) found slight variation in the characteristic sharp peaks of neem oil nanopreparations. These results indicate the absence of chemical interactions between nano-emulsion and the Na-Alg polymer. So, such a finding confirms that nano-emulsion was successfully encapsulated in Na-Alg polymer. In addition, the obtained results related to encapsulation efficiency (EE) and loading capacity (LC), similar data were obtained by (Lertsutthiworg, et al. [24]) who reported that (EE) and (LC) using distilled water were greater than that obtained by ethanol.
In the case of the results related to the acute oral LD50, it was observed a significant difference between the obtained LD50 values and the published ones. In other words, the determined LD50 values of the neem nano-formulation reached 113.33 and 134.83 mg/ kg body weight for loaded nano-emulsion and nano-emulsion, respectively while the LD50 value for the bulk formulation reached 140.90 mg/kg body weight. Such differences may be attributed to the small size of the prepared and/or loaded nanoparticles of neem oil which demonstrated a high surface area which lead to more penetration and hence exert their toxic effects through the produced reactive oxygen species (ROS) which affect in turn the integrity of all the cellular membranes of the targeted organs (Buzea, et al. [1]). However, various researchers reported that the bulk neem oil has no or low toxic effects on the treated animals. For example, (Bandyopadhyay, et al. [43]) stated that aqueous extract of Neem bark was found to be well-tolerated by both rats and mice (Boekea, et al. [44]). Reported that the non-aqueous extracts of neem appear to be the most toxic neem-based products, with an estimated safe dose (ESD) of 0.002 and 12.5 μg/kg BW/day. A similar finding was reported by (Raizada, et al. [17]) who found that the subchronic administration of azadirachtin did not produce any signs of toxicity, mortality changes in tissues weight, and serum of blood parameters.
At the biochemical level, the results related to the effect of the two tested neem nano-formulations compared to the bulk one at the sub-lethal doses (LD10) during continuous exposure for seven days on various biomarkers in the treated Swiss male albino mice, indicated that the mentioned effects may be attributed to the nanoformulations of neem rather than the bulk one. In other words, it is well established that the nanoparticle demonstrates a high chemical reactivity according to the quantum effect more than the bulk materials (Buzea, et al. [1]). Based on the necessity of toxicological information on the synthesized nanoparticles, several researchers have studied the toxic effects of different nanoparticles. For example, (Stigliani, et al. [45]) described the preparation and characterization of poly (ε-caprolactone) (PCL) nanocapsules loaded with neem oil, for possible use in pest control. An evaluation was made of their cytotoxic and genotoxic potentials. The aim was to obtain systems that were less harmful to the environment and human health and that could contribute to pest control in agriculture. The authors reported that in the case of the nanocapsules containing neem oil, a higher concentration of oleic acid and a lower concentration of azadirachtin could have resulted in higher toxicity. Although nanotechnology was still at an early stage in the agricultural sector, it was clear that there was growing interest in its use. The results obtained by (Stigliani, et al. [45]) showed that nano-capsules loaded with neem oil presented good colloidal stability and that all the nanoparticles were spherical. The cyto-, geno- and phytotoxicity studies showed that the two nano-capsules loaded with neem oil formulations, NC-10 formulation (100 mg of neem oil and 100 mg of oleic acid) presented the highest toxicity against non-target organisms, while the NC-20 formulation (200 mg of neem oil) presented the lowest toxic effect. They commented that these results may have been due to the interaction of components of neem oil with the oleic acid used in some formulations.
One of the good candidate biomarkers in toxicological studies is the hematological parameters which can be useful for the diagnosis of assessment of physiological status. Hemoglobin is an extremely essential part of red blood corpuscles that deliver oxygen to all parts of the body. Both low and high levels of this protein can adversely affect body health. In the present study, it was shown an increase in the hemoglobin (Hb) percent levels in some treatments on certain days during the administration (Scott, et al. [46]). Reported a significant increasing trend of (Hb) in stressed catfish (Ictalurus punctatus) leading to an elevated oxygen-carrying capacity of the individual erythrocytes. But in another trend, there were decreasing in (Hb) parameters on the fifth and seventh day in some neem formulation treatments. In this investigation, some treatments during certain days showed decreasing in hemoglobin percent levels and others showed increasing in comparison to the control animals and hemoglobin contents were paralleled with those of (RBCs). A similar finding was reported by (Athithya, et al. [47]) who found that ray-finned fish (Cirrhinus mrigala) treated with azadirachtin increased the level of (Hb) more than that of the control. In addition, (Johnson, et al. [48]) reported a significant reduction in the hematological parameters of animals treated with Azadirachta indica extract. They suggested that these reductions may be attributed to the cytotoxic effects and suppression of the erythropoiesis caused by constituents of the botanical extract.
However, (Parshad, et al. [49]) found that treatment of rats with aqueous neem leaf extract resulted in decreases in total bilirubin. Also, there were increases in packed cell volume (PCV), mean corpuscular hemoglobin (Hb) concentration, red blood corpuscles (to check the level of anemia), white blood cells, and lymphocyte counts, but no cytotoxic effects were observed. In another study carried out by (Abou-Tarboush, et al. [50]), it was found that azadirachtin was orally administrated to male and female SWR/J mice at a dose level of 9.0 mg/kg (1/10 LD50) for different treatment periods has produced signs of toxicity, mortality, and changes in the body, histopathological changes in the livers, kidneys and testis and tissue weights of both sexes at almost all treated periods. The present study showed a significant reduction in creatinine levels on certain days after treatment or recovery period as indicators for renal injury induced by neem formulation treatments. This effect may be due to its unique composition, where the neem oil is rich in flavonoids (rutin and quercetin, flavonol glycosides, polyphenols, and tannins). Flavonoids and polyphenols in neem oil have been described to possess both antioxidant and anti-inflammatory activities via scavenging free radicals and inhibiting lipid peroxidation (Chaisawangwong, et al. [51]).
The liver is the major organ for detoxification and removal of endogenous substances. It is continuously and widely exposed to hepatotoxins, xenobiotic substances, and chemotherapeutic agents that lead to impairment of its functions. So, the hepatotoxicity biomarkers were considered a key factor in the toxicity evaluation of the toxic agents. Based on such conception, the results of the investigated liver biomarkers in the treated mice indicated that the treatment with the tested neem bulk and nano-formulations affected such biomarkers significantly. Similar results were recorded by other researchers who found that the exposure to azadirachtin, both serum (AST) and (ALT) activities, and bilirubin content were increased significantly with accompanying high levels of lipid peroxidation and low levels of reduced glutathione (GSH) which an indicated to the hepatotoxicity of the liver in the animals administered with neem oil formulations. (Abdel Megeed, et al. [52- 54]). Conversely, (Kosoko, et al. [55]) reported that activities of liver and serum AST, ALT, and S-bilirubin of treated and untreated rats showed no significant changes in the clinico-chemical parameters of animals exposed to different concentrations of azadirachtin, and the values were found comparable to control.
In the case of the determined glutathione S-transferase (GST) activity and reduced glutathione (GSH) content, it was reported that GST catalyzes the conjugation of the xenobiotics with glutathione to facilitate the excretion of the toxic substances after its transformation of a water-soluble metabolite (Meister, et al. [56]). So, the recorded results related to the GST activity and GSH content in the treated mice were supported by (Moore, et al. [57]) who suggested that neem formulations are initially detoxified by conjugation with reduced glutathione (GSH) to form mercapturic acid (Arivazhagan, et al. [58]). Reported that a five-day pre-treatment of rats with leaf extract of neem decreased the formation of lipid peroxides and enhanced the levels of antioxidants and detoxifying enzymes in the stomach, liver, and circulation. Reduced glutathione (GSH) is an extremely efficient intracellular buffer for oxidative stress and (GSH) acts as a non-enzymatic antioxidant that reduces xenobiotics toxicity. In this investigation, it was shown significant variations between increasing and decreasing (GSH) levels in comparison with the control animals. Concentrations of (GSH) become very low on the other days in the administration of tested formulations and that could be referred to as the conjugation of liver (GSH) stores with the applied xenobiotic (neem formulations).
To investigate the effects of the tested neem formulations on the energy state of the treated mice was selected total ATPase as a biological marker which reflects its role in energy production in the tested animals. As reported by (Fogg, et al. [59]), the adenosine triphosphate (ATP), is well established that such a molecule is the source of energy for all living organisms. Such energy is released from the hydrolysis of the phosphate bonds in such a molecule by different forms of a key enzyme called (ATPases). These enzymes are essential for the transport of glucose, amino acids, etc. Any impairment in their activity will affect the physiology of the organism. The role of membrane lipids and their microenvironmental changes at the physical and chemical levels may be responsible for the differential response observed at the level of (ATPase) activity after treatment with neem extract. Membrane (ATPase) assists transport and reabsorption of metabolites and nutrients and also secondary transport of ions and nonelectrolytes. So that, the obtained results of alterations of the total ATPase activity after the first and third day of treatment by the neem formulation may be due to the changes in the mechanism of active ion transport in the cellular membranes and hence affect the membrane permeability of the treated mice which lead to alteration in electrolytic regulation. A similar finding was reported by several researchers (Babu, et al. [60-63]).
Conclusion
Characterization of the prepared nano-formulations of neem oil, i.e. nano-emulsion and loaded nano-emulsion revealed that the size of the prepared nanoparticles ranged between 30-80 nm. Based on the determined acute oral LD50 values, the nanoformulation exhibited higher mortality than the bulk one. The sub-acute administration of sub-lethal dose (LD10) showed significant alterations between induction and/or reduction in the hematological, hepatotoxicity, and nephrotoxicity biomarkers. Such alterations differed concerning the days after treatment of mice. More toxicological studies, i.e. chronic and long-term toxicity evaluations are required.
Ethical Approval
All the experiments using mice as experimental animals were carried out in compliance with the guidelines of the ethical principles in animal research adopted by the Ethics of Animal Use in Research Committee (EAURC), Egypt.
Disclosure Statement
No conflict of interest was reported by the authors.
References
- Buzea C, I I Pacheco, K Robbie (2007) Nanomaterials and nanoparticles: sources and toxicity. Biointerphases 2(4): 17-71.
- Bouwmeester H, S Dekkers, M Y Noordam, W I Hagens, A S Bulder, et al. (2009) Review of health safety aspects of nanotechnologies in food production. Regulatory Toxicology and Pharmacology 53(1): 52-62.
- Rajashekar Y, H Vijay Kumar, K V Ravindra, N Bakthavatsalam (2013) Isolation and characterization of biofumigant from leaves of Lantana camara for control of stored grain insect pests. Industrial Crops and Products 51: 224-248.
- Sharma S K, M Sai Ram (1996) Mechanism of action of NIM76: a novel vaginal contraceptive from neem oil. Contraception 54(6): 373-378.
- Gupta V K, S K Sharma (2006) Plants as natural antioxidants. Natural Product Radiance 5: 326-334.
- Balbus J M, A D Maynard, V L Colvin, V Castranova, G P Daston, R A Denison, et al. (2007) Meeting report: hazard assessment for nanoparticles—report from an inter disciplinary workshop. Environmental Health Perspectives 115(11): 1654-1659.
- Donaldson K, A Seaton (2007) The Janus faces of nanoparticles. Journal of Nanoscience and Nanotechnology 7(12): 4607-4611.
- Elsaesser A, C V Howard (2012) Toxicology of nanoparticles. Advanced Drug Delivery Reviews 64(2): 129-137.
- Shinde S K, N D Grampurohit, S L Gaikwad, S L Jadhav, M V Gadhave, et al. (2012) Toxicity induced by nanoparticles. Asian Pacific Journal of Tropical Disease 2(4): 331-334.
- Oberdorster G, A Maynard, K Donaldson, V Castranova, J Fitzpatrick, et al. (2005a). Principles for characterizing the potential human health effects from exposure to nanomaterials: elements of a screening strategy. Particle and Fibre Toxicology 2: 1-35.
- Lewinski N, V Colvin, R Drezek (2008) Cytotoxicity of nanoparticles. Small 4(1): 26-49.
- Oberdorster G, V Stone, K Donaldson (2007b) Toxicology of nanoparticles: A historical perspective. Nanotoxicology 1(1): 331-341.
- Dimetry N Z (2012) Prospects of botanical pesticides for the future in integrated pest management program (IPM) with special reference to neem uses in Egypt. Archives of Phytopathology and Plant Protection 45: 1138-1161.
- Dimetry N Z, A Y El-Laithy, A M E Abd El-Salam, A E El-Saiedy (2013) Management of the major piercing-sucking pests infesting cucumber under plastic house conditions. Archives of Phytopathology and Plant Protection 46(2): 158-171.
- Mubayi A, S Chatterji, P M Rai, G Watal (2012) Evidence based green synthesis of nanoparticles. Advanced Materials 3(6): 519-525.
- (1992) Anonymous. Neem: a tree for solving global problems, Report of an Ad Hoc Panel of the Board on Science and Technology for International Development, National Research Council. Pp: 60-113. National Academy Press, Washington D.C., USA.
- Raizada R B, M K Srivastava, R A Kaushal, R P Singh (2001) Azadirachtin, a neem biopesticide: Subchronic toxicity assessment in rats. Food and Chemical Toxicology 39(5): 477-483.
- Niemann L, V Hilbig (2000) Neem seeds extract as an example for health evaluation of naturally occurring substances to be applied in plant protection. Gesunde Pflanzen 52(5): 135-141.
- Wang B, W Y Feng, M Wang, T C Wang, Y Gu, et al. (2008) Acute toxicological impact of nano- and submicro-scaled zinc oxide powder on healthy adult mice. Journal of Nanoparticle Research 10: 263-276.
- Kong T, S Zhang, J Zhang, Z Hao, F Yang, et al. (2018) Acute and cumulative effects of unmodified 50-nm nano-ZnO on mice. Biological Trace Element Research 180: 124-134.
- Bray DH, D C Warhurst, J D Connolly, M J O’Neill, J D Phillipson, et al. (1990) Plants as sources of antimalarial drugs. Part 7. Activity of some species of Meliaceae plants and their constituent limonoids. Phytotherapy Research 4: 29-35.
- Jerobin J, R S Sureshkumar, C H Anjali, A Mukherjee, N Chandrasekaran (2012) Biodegradable polymer-based encapsulation of neem oil nanoemulsion for controlled release of Aza-A. Carbohydrate Polymers 90(4): 1750-1756.
- Bouchemal K, S Briançon, E Perrier, H Fessi (2004) Nano-emulsion formulation using spontaneous emulsification: solvent, oil and surfactant optimization. International Journal of Pharmaceutics 280(1-2): 241-251.
- Lertsutthiwong P, K Noomun, N Jongaroonngamsang, P Rojsitthisak, U Nimmannit, et al. (2008) Preparation of alginate nanocapsules containing turmeric oil. Carbohydrate Polymers 74(2): 209-214.
- Rivera M C, A C Pinheiro, A I Bourbon, M A Cerqueira, A A Vicente, et al. (2015) Hollow chitosan/alginate nanocapsules for bioactive compound delivery. International Journal of Biological Macromolecules 79: 95-102.
- Xue J, Z Zhang (2007) Encapsulation of peppermint oil for Dental care. Proceeding of XVth workshop on Bioencapsulation Vinnie, p. 1-4.
- Kumar S, G Bhanjana, A Sharma, M C Sidhu, N Dilbaghi, et al. (2014) Synthesis, characterization and on-field evaluation of pesticide loaded sodium alginate nanoparticles. Carbohydrate Polymers 101: 1061-1067.
- Weil C S (1952) Tables for convenient calculation of median-effective dose (LD50 or ED50) and instructions in their use. Biometrics 8(3): 249-263.
- Hayes W J (1975) Handbook of Pesticide Toxicology: Dosage and Other Factors Influencing Toxicity. Wavely press: USA.
- Drabkin D L, J H Austin (1932) Spectrophotometric constants for common hemoglobin derivatives in human, dog and rabbit blood. Journal of Biological Chemistry 98: 719-733.
- Reitman A, S Frankel (1957) A colorimetric method for the determination of serum glutamic oxalacetic and glutamic pyruvic transaminases. American Journal of Clinical Pathology 28(1): 56-63.
- Habig W H, M J Pabst, W B Jakoby (1974) Glutathione S-Transferases the first enzymatic step in mercapturic acid formation. Journal of Biological Chemistry 249(22): 7130-7139.
- Ellman G L (1959) Tissue Sulfhydryl Groups. Archives of Biochemistry and Biophysics 82(1): 70-77.
- Walter M, H Gerade (1970) A colorimetric method for determination bilirubin in serum and plasma. Microchemical Journal 15: 231-236.
- Tobias G J, R F McLaughlin, J Hopper (1962) Endogenous Creatinine Clearance — A Valuable Clinical Test of Glomerular Filtration and a Prognostic Guide in Chronic Renal Disease. The New England Journal of Medicine 266: 317-323.
- Sarkadi B, E M Prince, R C Boucher, U A Germann, G A Scarborough, et al. (1992) Expression of the human multidrug resistance cDNA in insect cells generates a high activity drug-stimulated membrane ATPase. Journal of Biological Chemistry 267(7): 4854-4858.
- Bradford M M (1976) A rapid and sensitive method for the quantitative of microgram quantities of protein utilizing the principle of protein-dye binding. Analytical Biochemistry 72: 248-254.
- Ghotbi R S, M Khatibzadeh, S Kordbacheh (2014) Preparation of neem seed oil nanoemulsion. Proceedings of the 5th International Conference on Nanotechnology: Fundamentals and Applications Prague, Czech Republic, August, p. 11-13.
- Kale N J, L V Allen (1989) Studies on microemulsions using Brij 96 as surfactant and glycerin, ethylene glycol and propylene glycol as cosurfactants. International Journal of Pharmaceutics 57(2): 87-93.
- Karthikeyan S, P A Jeeva, J Jerobin, A Mukherjee, N Chandrasekaran (2012) Formulation and characterization of nanoemulsion coatings from Azadirachta indica. International Journal of ChemTech Research 4(4): 1566-1570.
- Kulkarni A R, K S Soppimath, T M Aminabhavi, A M Dave, M H Mehta, et al. (2000) Glutaraldehyde cross-linked sodium alginate beads containing liquid pesticide for soil application. Journal of Controlled Release 63(1-2): 97-105.
- Siddiqui B S, M Rasheed, F Ilyas, T Gulzar, R M Tariq, et al. (2004) Analysis of insecticidal Azadirachta indica A. Juss. Fractions. Zeitschrift fur Naturforschung 20 (1-2): 104-112.
- Bandyopadhyay U, K Biswas, R Chatterjee, D Bandyopadhyay, I Chattopadhyay, et al. (2002) Gastroprotective effect of neem (Azadirachta indica) bark extract: Possible involvement of H+-K+-ATPase inhibition and scavenging of hydroxyl radical. Life Sciences 71(24): 2845-2865.
- Boekea S J, M G Boersmab, G M Alinkb J J A vanLoona, A VanHuisa, M Dickea, et al. (2004) Safety evaluation of neem (Azadirachta indica) derived pesticides. Journal of Ethnopharmacology 94(1): 25-41.
- Stigliani T P, V R Estefânia, J L Campos, C M G Oliveira, N B J Silva, et al. (2017) Nanocapsules Containing Neem (Azadirachta indica) Oil: Development, Characterization and Toxicity Evaluation. Scientific Reports 7(1): 5929-5931.
- Scott A L, W A Rogers (1981) Hematological effects of prolonged sublethal hypoxia on channel catfish Ictalurus punctatus (Rafinesque). Journal of Fish Biology 18(5): 591-601.
- Athithya N, S Anbazahana R Nithyaa, M N Ranib C Balasundaramb, R Harikrishnana (2014) Effect of azadirachtin on hematological and biochemical parameters of Cirrhina mrigala infected with Aphanomyces invadan. Proceedings of the 7th National Symposium on Advance Research in Biosciences, 3rd & 4th March, 2014, Bharath College of Science and Management, Thanjavur, Tamil Nadu, India.
- Johnson M, L A Olufunmilayo, D O Anthony, E O Olusoji (2015) Hepatoprotective Effect of Ethanolic Leaf Extract of Vernonia amygdalina and Azadirachta indica against Acetaminophen-Induced Hepatotoxicity in Sprague-Dawley Male Albino Rats. American Journal of Pharmaceutical Sciences 3(3): 79-86.
- Parshad O, P Singh, M Gardner, C Fletcher, E Rickards, et al. (1994) Effects of aqueous neem (Azadirachta indica) extract on testosterone and other blood constituents in male rats. West Indian Medical Journal 43(3): 71-74.
- Abou-Tarboush F M, H M El-Ashmaoui, H I Hussein D Al-Rajhy, M Al-Assiry, et al. (2009) Effect of azadirachtin of neemix-4.5 on SWR/J mice. Saudi Journal of Biological Sciences 16(2): 69 -76.
- Chaisawangwong W, W Gritsanapan (2013) Quality assessment and scavenging activity of Siamese neem flower extract. Natural Product Research 27(4-5): 394-401.
- Abdel Megeed M I, U M Radwan, A Z Hindy, A El Zarook (2001) Liver functions under stress of certain common pesticides residue used on fruits and vegetables orally administrated. Annals of Agricultural Sciences 46: 383-404.
- Srivastava M K, R B Raizada (2005) Lack of toxic effect of technical azadirachtin during postnatal development of rats. Food and Chemical Toxicology 45(3): 465-471.
- Mahesh A, J Shaheetha, D Thangadurai, D M Rao (2009) Protective effect of Indian honey on acetaminophen-induced oxidative stress and liver toxicity in rat. Biologia 64: 1225-1231.
- Kosoko A M, O J Olurinde, C A Leigh, O T Somade, R O Akanbi, et al. (2018) Potential Genotoxicity, Hematotoxicity and Clastogenicity of Three Commonly Consumed Polyherbal Formulations in Abeokuta South-West Nigeria. International Journal of Chemical and Biomedical Science 4(2): 34-45.
- Meister A R, M E Anderson (1983) Glutathione. Annual Review of Biochemistry 52: 711-760.
- Moore M, H Thor, G Moore, S Nelson, P Moldeus, et al. (1985) The toxicity of acetaminophen and N-acetyl-p-benzoquinone imine in isolated hepatocytes is associated with thiol depletion and increased cytosolic Ca2+. Journal of Biological Chemistry 260(24): 13035-13040.
- Arivazhagan S, S Balasenthil, S Nagini (2000) Modulatory effects of garlic and neem leaf extracts on N-methyl-N-nitro-N-nitrosoguanidine (MNNG)-induced oxidative stress in Wistar rats. Cell Biochemistry and Function 18(1): 17-21.
- Fogg K, J Anstee, D Hyde (1991) Studies on the subcellular distribution of (Na++ K+)-ATPase, K+-stimulated ATPase and HCO3 (-)-stimulated ATPase activities in Malpighian tubules of Locusta migratoria L. Insect Biochemistry 21(7): 749-758.
- Babu R, K Murugan, G Vanithakumari (1996) Interference of Azadirachtin on the food utilization efficiency and mid gut enzymatic profiles of Helicoverpa armigera. Indian Journal of Environment and Toxicology 6: 81-84.
- Rahman MF, M K Siddiqui, K Jamil (1999) Sub-chronic effect of neem-based pesticide (vepacide) on acetylcholinesterase and ATPase in the rat. Journal of Environmental Science and Health, Part B 34 (5): 873-884.
- Nathan S S, K Kalaivani, P G Chung (2005) The effect of azadirachtin and nucleopolyhedrovirus on midgut enzymatic profile of Spodoptera litura Fab. (Lepidoptera: Noctuidae). Pesticide Biochemistry and Physiology 83(1): 46-57.
- Fiske CH, Y Subbarow (1925) The Determination of Phosphorus and the discovery of phosphocreatine and ATP. Journal of Biological Chemistry 81: 629- 679.