Abstract
Oxidative stress is known by excess production of reactive species, especially (ROS) and deleterious modifications in biomolecules such as proteins, and lipid mainly. It is a common mediator in pathogenesis of multiple diseases. Because of its potential to act as center in the cause of many diseases particularly cardiovascular disease, identifying biomarkers of oxidative stress has become the highly interesting and focus of many research. Measurement of reactive species (ROS) in the circulation of complex biological systems remains a challenge due to the short half-life of these reactive species and the need of special equipment to detect it. A widely used and reliable approach is the measurement of stable by-products modified under conditions of oxidative stress that have entered the circulation. The stable oxidation product that used as biomarker of oxidative stress include lipid oxidation product such as Isoprostanes (isops), MDA, and protein oxidation product such as nitrotyrosine ,Protein Glutathionilation and oxd LDL are the major one. The oxidative stress play pivotal role in the pathogenesis of the major cardiovascular risk factors termed as atherosclerosis. This is caused by excess production of reactive species (ROS) in the vessel mainly by enzymes such as NAD (P)H oxidase, xanthine oxidase, myeloperoxidase and uncoupled nitrc oxide synthase which oxidize lipoprotein (LDL). The oxidize LDL (oxLDL) lipoprotein progress the atherosclerosis process from the initially to the end stage development.
Keywords: Oxidative Stress; OxLDL; Atherosclerosis; Reactive Species
Abbreviations: CVD: Cardiovascular Disease; DNA: Deoxyribonucleic acid: ELISA: Enzyme-linked Immunosorbance Assays; eNOS: Endothelial Nitric Oxide Synthase; GC: Gas Chromatography; ICAM-1: Intercellular Adhesion Molecule-1; LC: Liquid Chromatography; LDL: Low-Density Lipoproteins; MCP-1: Monocyte Chemotactic Protein-1; M-CSF: Macrophage Colony-Stimulating Factor; MDA: Malondialdehyde; MS: Mass Spectrometry; nNOS: Neuronal Nitric Oxide Synthase cells; OxLDL: Oxidized Low Density Lipoprotein; Phox: Phagocyte Oxidase; PUFAs: Polyunsaturated Fatty acids; RNS: Reactive Nitrogen Species; ROS: Reactive Oxygen Species; SMC: Smooth Muscle Cells; SRs: Scavenger Receptors; TBA: Thiobarbitoric acid; VCAM-1: Vascular Cell Adhesion Molecule-1; XOR: Xanthine Oxidoreductase; XO: Xanthine Oxidase
Introduction
Nowadays, Oxidative Stress (OS) has increasingly become a major interesting area of basic science and clinical research. It is the imbalance between generations and clearances of oxidants as shown in (Figure 1). Oxidants are composed of reactive free radical and radical such as Reactive Oxygen Species (ROS) and Reactive Nitrogen Species (RNS). ROS are composed of superoxide radical (O2 -), hydroxyl radical (•OH), and hydrogen peroxide (H2O2). RNS include Nitric Oxide (NO), Nitrogen Dioxide (NO2), and peroxynitrite (ONOO•). These are involved in the modification of several macromolecules such as lipid, protein, DNA and causing deleterious effects in several organs [1,2]. Oxidants removed through antioxidant defense mechanism under normal physiological condition. The incomplete removals of oxidants by antioxidants lead to its accumulation and generate oxidative stress condition. This is the result of biochemical processes leading to the production of excess reactive species and limited capabilities of endogenous antioxidant systems. Oxidizing agents (reactive species) can be produced by both endogenous source such as inflammatory cells, fibroblast, epithelial cells, endothelial cells, respiratory chain, xanthine and NADPH oxidase and exogenous source such as cigarette smoke, unhealthy diet, exogenous toxins, pollution, radiation [3,4]. Research has revealed a widespread involvement of oxidative stress in a number of disease processes such as cancer, Cardiovascular Disease (CVD), atherosclerosis, diabetes, arthritis, neurodegenerative disorders, and pulmonary, renal, and hepatic diseases during the past decade, [5-9]. Therefore, identifying biomarkers of oxidative stress are becoming important area of research in the recent years.
Biomarker is characteristic that is objectively measured and evaluated as an indicator of normal biological processes, pathogenic processes, or pharmacological responses to a therapeutic intervention. Biomarkers of oxidative stress are also important to assess the biological redox status, disease state and progression, and the health enhancing effects of antioxidants in humans. Measurement of reactive species such as ROS, RNS in the circulation of complex biological systems as biomarker of oxidative stress remains a challenge due to the short half-life of these reactive species and the need of special equipment to detect. A popular approach for assessing biomarker of oxidative stress is the measurement of stable by-products modified under conditions of oxidative stress by excess reactive species and that have entered the circulation. These can be classified by molecules that are modified by interactions with ROS in the microenvironment. Molecules that can be modified by excessive ROS include DNA, lipids (including phospholipids), proteins and carbohydrates. Some of these modifications have a direct effect on the function of target molecules, such as the inhibition of an enzymatic function, but other modifications just reflect the local degree of oxidative stress [6,7].
Biomarkers Based on Ros-Induced Modifications
Lipid Peroxidation Product
Lipid peroxidation is reaction of oxygen with unsaturated lipids and produces a wide variety of oxidation products. A main lipid peroxidation product includes isoprostanes and aldehydes like Malondialdehyde (MDA). Determination of these lipid peroxidation products used as oxidative stress biomarker
Isoprostanes
The Isoprostanes (IsoPs) are bioactive prostaglandin-like compounds formed via a nonenzymatic mechanism through the free radical-initiated peroxidation of arachidonic acid [3,4]. Sources of free radicals for IsoPs formation include mitochondrial electron transport chain, P450 enzymes, lipoxygenase, and transition-metal catalyzed formation of free radicals. The F2-IsoPs are the most chemically stable and reliable marker for assessing oxidative stress status from the IsoPs family. It is called F2-IsoPs because they contain F-type prostane rings. Initially formed in lipid membranes as a consequence of oxidative stress and then released in free form by phospholipase action into circulation [10,11]. Elevated F2-IsoPs are found in a wide range of human clinical conditions. Elevated concentrations of F2-IsoPs are found in CVD, correlate with extent of disease, and predict the outcome [12,13]. IsoPs measured using most reliable methods such as combined Gas Chromatography- Mass Spectrometry (GC-MS) and Liquid Chromatography-Mass Spectrometry (LC-MS) in plasma and urine samples. Because of these methods are labor-intensive, require specialized and expensive instrumentation, it becomes challenge to widespread adaptation of F2-IsoPs in clinical trials [14].
Malondialdehyde
Malondialdehyde (MDA) is one of the aldehydes products of enzyme and free radical which can be formed as secondary lipid peroxidation products during lipid peroxidation. MDA is generated by decomposition of arachidonic acid and larger Polyunsaturated Fatty Acids (PUFAs). It is chemically more stable and membranepermeable than ROS and less toxic than other secondary lipid peroxidation products [15]. MDA is the most frequently used oxidative stress biomarker in many health problems such as cancer, psychiatry, chronic obstructive pulmonary disease, asthma, or cardiovascular diseases. Thiobarbitoric Acid (TBA) assay is the most commonly used method for determination of the MDA in biological fluids. Free and total MDA also determined using several technologies such as Gas Chromatography (GC), Mass Spectrometry (MS), Liquid Chromatography (LC) [16,17].
Protein Oxidation Products
Proteins represent a wide target for ROS and RNS generated under normal or oxidative stress conditions and can be considered as general scavengers of these species. Several amino acidic residues can undergo oxidative modification the major one include oxidation of sulphur-containing residues, nitration of tyrosine residues, and glutathionylation of cysteine residues [18]. Nitrotyrosine: Nitrotyrosine (Tyr-NO2) is a stable oxidative stress biomarker in inflammatory diseases. It occurs by several pathways but all involves RNS and a two-step tyrosine nitration process. One widely studied pathway for nitration is mediated by reactive nitrogen species such as peroxynitrite (ONOO) and nitrogen dioxide (NO2). Thus, Tyr-NO2 was initially believed to be a specific marker of peroxynitrite-mediated damage [19]. The twostep process are
a) Tyrosine is oxidized resulting in a tyrosine radical and
b) A radical–radical reaction occurs between the tyrosine radical and nitrogen dioxide (NO2).
Finally, Tyr- NO2, result from the replacement of C3 hydrogen atom of the tyrosine aromatic ring with a nitro group (R- NO2) as shown in (Figure 2). This modification can occur within a polypeptide sequence (protein-associated Tyr- NO2) or to free tyrosine amino acids (free Tyr- NO2). Studies showed that 3-NO-Tyr plasma levels are increased in several conditions such as asthma, diabetes, and cardiovascular diseases, and reduced following therapeutic treatments [20].
Oxidized Low-Density Lipoprotein: Low-Density Lipoproteins (LDL) can undergo oxidative modification. The oxidation of LDL can occur non-enzymatically or enzymatically. MDA’s reaction with lysine residues generates lysine–lysine crosslinks which have been identified in apolipoprotein B (apoB) fractions of oxidized low density lipoprotein (OxLDL). These enhance the interaction between OxLDL and macrophages which in turn promote atherosclerosis and cardiovascular diseases [21,22]. OxLDL levels are higher in patients with CVD and increasing its levels correlate with increasing severity of disease. OxLDL levels also appear to be predictive of future CAD in apparently healthy men. Plasma oxLDL has also been consistently found elevated in patients with diabetes and obesity. The most common test makes use of specific antibodies that recognize selected modifications of LDL amino acidic residues. OxLDL is most commonly detected in plasma or isolated LDL by using specific monoclonal antibodies that directly recognize unique oxidation specific epitopes. One of three different antibodies that are available for immunologic assay (ELISA) of OxLDL in research purposes includes: 4E6, DLH3, and E06. The monoclonal antibody, 4E6, binds to aldehyde-modified lysine residues on LDL epitope. The monoclonal antibodies, DLH3 and E06, recognize oxidized phosphatidylcholine and phosphorylcholine containing short oxidized or nonoxidized side chains, respectively [23].
Protein Glutathionilation: Reversible protein-S- glutathionylation can occur under physiological conditions within as result of GSH antioxidant activity. Cysteine- (SOH-) glutathionilation (S-Glutathionylation) formed through the formation of a disulphide bridge between a reactive cysteine residue and the abundant cellular tripeptide glutathione. This oxidative modification can exert effects on protein tertiary structure and function in a manner similar to phosphorylation [24]. It has been shown to mediate redox regulation of a number of key cellular proteins including Endothelial Nitric Oxide Synthase (eNOS), SERCA and Na+ -K+ pump [25] as shown in (Figure 3). The impact of glutathionylation of each of these membrane proteins has been reported in either the myocardium or vascular tissue with altered function resulting in alterations in intracellular Na+and Ca2+. However, the direct usefulness of measuring glutathionylation of these proteins as biomarkers is hampered by difficulty in accessing the tissue in which these functionally relevant modifications occur. S-glutathionylation of susceptible proteins is commonly measured using Western Blotting [24,26].
The Role of Oxidative Stress in Atherosclerosis Development
Oxidative stress has a pivotal role in the progression of atherosclerosis which linked to cardiovascular disease. According to the theory of oxidative stress, atherosclerosis is the result of the oxidative modification of Low Density Lipoproteins (LDL) in the arterial wall by Reactive Oxygen Species (ROS). ROS initiate several processes involved in atherogenesis such as expression of adhesion molecule, stimulation of vascular smooth muscle proliferation and migration, apoptosis in the endothelium, oxidation of lipids, activation of matrix metalloproteinases, and altered vasomotor activity. The reactions of O2 - with NO produce peroxynitrite which is less effective for the activation of guanilyl cyclase. Subsequently, NO bioavailability becomes remarkably reduced. Oxidative stress (H2 O2) affects atherogenesis through the production of transcription factors such as nuclear factor and activator protein 1 (AP-1), which participate in the expression of adhesion molecules, such as vascular cellular adhesion molecules (VCAM-1), intracellular adhesion molecules (ICAM-1), E-selectin and other cytokines [27,28]. Therefore, there has been particular interest in the enzyme sources that contribute to the production of free radicals (ROS) in the vessel wall. Enzymes generating ROS are involved in several cell functions and their alteration may result in imbalanced redox status. The important enzyme sources responsible for ROS production include NAD (P) H oxidase, Xanthine Oxidase (XO), Myeloperoxidase (MPO), and uncoupled form of Nitric Oxide Synthase (NOS) [29] as shown in (Figure 4). Some ROS-generating enzymes can be found in the circulation and thus can be used as oxidative stress markers.
Main Ros Producing Enzymes in Atherosclerosis
Nicotinamide-Adenine Dinucleotide Phosphate-Oxidase
NAD (P) H oxidase is a membrane-bound enzyme complex which represents a major source of O2 - in the body. It is present in various cells such as the endothelial cells, smooth muscle cells, fibroblasts, monocytes, and macrophages. The NAD(P)H oxidase found in neutrophils has five subunits: p22phox, p47phox, p67phox , p40phox (phox stands for phagocyte oxidase), and the catalytic subunit gp91phox. p22phox and gp91phox are present in the membrane whereas p47phox, p67phox, and p40phox exist in the cytosol. A number of stimuli activate NAD (P) H oxidase whereby p47phox becomes phosphorylated and the cytosolic subunits form a complex that translocates to the membrane and convert the oxidase into active form which transfers electrons from the substrate to O2, forming O2-[30]. In the first step one electron is added to the molecular oxygen in a univalent reduction to generate superoxide anion O2 - using NADPH as the electron donor:

Xanthine Oxidase
Xanthine Oxidoreductase (XOR) is another important enzymatic source of ROS which belongs to metalloflavoprotein family. It catalyzes the oxidation of hypoxanthine and xanthine to form uric acid. XOR exists in two interconvertible forms: Xanthine Oxidase (XO) and Xanthine Dehydrogenase (XDH). XO oxidizes xanthine to UA using oxygen as the electron acceptor and produces superoxide or H2 O2 while XDH carries out the same reaction but uses NAD+ and generates NADH [31]. Most of the circulating XOR form exists in the oxidase form. The enzyme catalyzes the reduction of O2 and formation of superoxide O2 - and H2 O2 which proposed as a central mechanism of oxidative injury. Principle reaction catalyzed by Xanthine Oxidase (XO) is the oxidation of xanthine into uric acid:

This process is accompanied by production of superoxide:
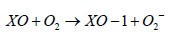
Role of XO in Atherosclerosis Development: Upregulation of XO activity may lead to an increase in UA serum levels, oxidative stress, and endothelial dysfunction [32]. The concentration of circulating XOR is low under normal physiological conditions, but it increases dramatically in certain diseases. Inflammation promotes XDH expression in vascular endothelial cells and stimulates XDH release into the circulation. Once in the circulation XDH is quickly converted, by reversible oxidation of the sulfhydryl residue or by irreversible proteolysis into XO which binds to the endothelial surface, resulting in amplified XO-derived ROS formation. Oxidants produced by endothelium-bound XOR have been implicated in the pathogenesis of atherosclerosis by a variety of studies. XO-induced oxidative stress has detected in cardiovascular diseases such as heart failure [33].
Nitric Oxide Synthase
There are three known isoforms of NOS with different activities. Two of the NOS isoforms are expressed constitutively these are in neuronal cells (nNOS) and endothelial cells (eNOS). Another isoform of NOS which is expressed in macrophages when needed termed as induced (iNOS) [30]. The NOS have emerged as important sources of superoxide, particular the endothelial isoform of NOS (eNOS) in the last few years. These enzymes use tetrahydrobiopterin (BH4) as a cofactor for transfer of electrons from a heme group within the oxygenase domain to L-arginine to form L-citrulline and NO under normal conditions. If either BH4 or L-arginine is absent, the electrons from heme reduce oxygen to form superoxide. Conditions such as hypercholesterolemia, Diabetes Mellitus (DM), arterial hypertension, smoking, age, and nitrate intolerance associated with NOS uncoupling through increasing the production of ROS. When low-level ROS are generated, oxidation of BH4 lead to diminished amount of BH4 but increased amount of oxidized BH4. These can then lead to a marked increase in superoxide from the NOSs [27,28].
Conversion of l-arginine to l-citrulline and nitric oxide is carried out by NOS:

But under uncoupling conditions, these enzymes also produce superoxide:

Role of Nos in Atherosclerosis Development: Impairment of the NOS isoform-dependent pathways is one of the earliest events in atherogenesis. CHD risk factors that deplete levels of L-arginine or BH4 may promote NOS-mediated ROS formations which in turn increase peroxynitrite generation. Decreased NO bioavailability disrupts the non-thrombogenic intimal surface and promotes platelet adhesion and aggregation as well as deposition of platelets on the abnormal endothelial surface [34,35] (Figure 5).
Myeloperoxidase
Myeloperoxidase (MPO) is a heme containing peroxidase synthesized and secreted by neutrophils and monocytic cells is an important source of ROS. Its physiological function is to utilize the H2 O2 produced by membrane-associated NADPH oxidase to induce lipid peroxidation at a site of inflammation. MPO can also catalyze the formation of hypohalous acids such as HOCl and/or HOBr using H2 O2 and Br-/Cl- [36]. ROS and reactive halogenating species modify various biomolecules such as nucleic acids, prozteins, and lipids. MPO also plays an essential role in host immune defenses because of its unique ability to generate HOCl, which has potent antimicrobial activity as shown in (Figure 6).
Role of Myeloperoxidase in Atherosclerosis Development: Many studies implicated MPO in the pathogenesis of atherosclerosis, showing that it is enriched within atheromatous plaques. Inflammatory cells recruited into the vascular wall release MPO-derived ROS that can in turn generate atherogenic OxLDL and modify High Density Lipoprotein (HDL), impairing its function in cholesterol efflux [38]. MPO high levels in the circulations are associated with cardiovascular disease [39]. MPO has emerged as one enzymatic catalyst for LDL oxidation via several chemical processes. MPO forms the highly reactive HOCl, which preferentially reacts with amino acids rather than lipids. HOCl chlorinates electron-rich substrates on apolipoprotein B-100 such as Lys residues and Tyr forming MPO-specific 3- chlorotyrosine. Enrichment of LDL with markers of chlorination such as 3-chlorotyrosine, served to identify MPO as the first enzymatic catalyst of a specific oxidative pathway [40,41]. In addition, one MPO’s role to atherosclerosis involves site specific oxidative modification of HDL, thereby attenuating its antiatherogenic properties. MPO binds to helix 8 on apolipoprotein A-I, the major protein associated with HDL. In the presence of H2 O2, Cl- and/or NO2 -, MPO may decrease the ability of HDL to facilitate cholesterol efflux from lipid-laden cells [42-44]. Oxidized HDL competes with native HDL as a ligand for the scavenger receptor, and this may further interfere with mobilization of cholesterol from peripheral tissues to the liver. It also resulted in proinflammatory particle that induced the expression of vascular cell adhesion molecule 1 in endothelial cells, and hence may promote the entry of circulating monocytes into the vessel wall [45,46] (Figures 7 & 8).
The Role of Oxidized Ldl in Atherosclerosis Development
The oxidation of lipoproteins is an initial phase in the development of atherosclerosis with deleterious and toxic effects on endothelial cells [48]. One of lipoproteins oxidation product ,Ox- LDL, induce endothelial dysfunction, the expression of adhesion molecules, the migration and proliferation of smooth muscle cells, and foam cell formation [49]. Atherosclerosis has three important stages heralded by a fatty streak formation, the induction of atheroma, and atherosclerotic plaques which eventually lead to atherothrombosis [50]. Low-Density Lipoprotein (LDL-C) penetrates into the vascular intima through certain risk factors and, it accumulates in the intimal layer of the arteries. Then, ROS convert the LDL-C to reactive Ox-LDL, and these remain in the vascular intima [51-53]. The Oxidized Lipids (Ox-LDL) induce the activation of endothelial cells and the activated endothelial cells release proinfammatory factors such as cytokines. Cytokines includes tumor necrosis factor (TNF-α), interleukin(IL-1, IL-4, IL-6) and interferon gamma (IFN-γ) which induce the expression of monocyte adhesion molecules like Vascular Cell Adhesion Molecule-1 (VCAM), Intercellular Adhesion Molecule-1 (ICAM) and E-selectin on the endothelial surface and these inturn lead to accumulation of monocytes in the vascular wall intima [49-52] .
Monocytes in the sub-endothelial space are differentiated into macrophages through chemotactic proteins such as Monocyte Chemotactic Protein-1 (MCP-1), Macrophage Colony-Stimulating Factor (M-CSF), and IL-8. Macrophages recognize and uptake Ox-LDL molecules via “Scavenger” Receptors (SRs). Finally, macrophages become foam cells, and the aggregation of yellow foam cells on the arterial walls leads to development of fatty streaks. A necrotic core is formed as the result of apoptotic foam cell death, and this acts as storage for cellular debris and lipids [54]. A fibrous atherosclerotic plaque cap is created during the migration of smooth muscle cells from media to intima vascular layers and the induction of extracellular matrix production in atheroma formation. The atherosclerotic plaque cap consists of collagenrich fiber tissues, Smooth Muscle Cells (SMC), and macrophages. Atheromatous lesions are developed by tissue macrophages and decreased blood flow in the vessels [52]. Macrophages and T lymphocytes secrete metalloproteinase and TNF-α in the margins of the developing plaque in order to lyse the fibrous cap extracellular matrix and inhibit collagen synthesis in the SMC, respectively. The lysis of the extracellular matrix leads to the destruction of the fibrous cap, and the thrombogenic contents are exposed to the blood stream initiating the coagulation process, blood clot formation, the adhesion of platelets, and thrombus formation, which may completely block the arteries [55-57] (Figure 9).
Summary
Oxidative stress biomarkers like other biomarker used as indicator of normal biological processes, pathogenic processes, or pharmacological responses to a therapeutic intervention. The parallel use of multiple biomarkers of oxidative stress could increase the reliability of the biomarker in diagnosis and prognosis of disease. The use of oxidative stress biomarkers play pivotal role in guiding pharmacological and non-pharmacological (natural antioxidant) therapeutic interventions. The using of oxidative stress biomarkers in the clinical and experimental area is the highly demanding one however the widespread use of it is challenged by the nature of the biomarker (reactivity), the expensive laboratory instrument requirement, labor intensive and the care for their laboratory process are the major one. Oxidative stress play crucial role in the development of the several diseases particularly the cardiovascular disease risk factor is the major one (atherosclerosis). The vascular ROS producing enzymes play important role in inducing oxidative stress condition that elicit and progress the atherosclerosis development (Figures 10 & 11).
Competing Interests
The authors declare that they have no competing interests.
Authors Contribution
ET, GB and CKE designed the study, analyzed and interpreted the data and contributed to manuscript writing. Both authors read and approved the final manuscript.
References
- Rác M, Křupka M, Binder S, Sedlářová M, Matušková Z (2015) Oxidative damage of U937 human leukemic cells caused by hydroxyl radical results in singlet oxygen formation. PLoS ONE 10(3).
- Matsubara K, Higaki T, Matsubara Y, Nawa A (2015) Nitric oxide and reactive oxygen species in the pathogenesis of preeclampsia. International Journal of Molecular Sciences 16(3): 4600-4614.
- Ho E, Galougahi KK, Liu C, Bhindi R, Figtree GA (2013) Biological markers of oxidative stress : Applications to cardiovascular research and practice. Redox Biol 1(1): 483-491.
- Marrocco I, Altieri F, Peluso I (2017) Measurement and clinical significance of biomarkers of oxidative stress in humans. Oxidative medicine and cellular longevity 9(10): 78-109.
- Palipoch S, Koomhin P (2015) Oxidative stress-associated pathology: a review. Sains Malaysiana 44(10): 1441-1451.
- A Negre Salvayre, N Auge, V Ayala (2010) Pathological aspects of lipid peroxidation. Free Radical Research 44(10): 1125-1171.
- DN Granger, PR Kvietys (2015) Reperfusion injury and reactive oxygen species: the evolution of a concept. Redox Biology 6:524-551.
- CC Winterbourn (2014) The challenges of using fluorescent probes to detect and quantify specific reactive oxygen species in living cells. Biochimica et Biophysica Acta 1840(2): 730-738.
- SI Dikalov, DG Harrison (2014) Methods for detection of mitochondrial and cellular reactive oxygen species. Antioxidants & Redox Signaling 20(2): 372-382.
- U Dreissigacker, MT Suchy, N Maassen, D Tsikas (2010) Human plasma concentrations of malondialdehyde (MDA) and the F2-isoprostane 15(S)-8-iso-PGF(2alpha) may be markedly compromised by hemolysis: evidence by GC-MS/MS and potential analytical and biological ramifications. Clinical Biochemistry. 43(1-2): 159-167.
- KA Smith, J Shepherd, A Wakil, ES Kilpatrick (2011) A comparison of methods for the measurement of 8-isoPGF (2alpha): a marker of oxidative stress. Annals of Clinical Biochemistry 48(2): 147-154.
- Davies SS, Roberts LJ (2011) F2-isoprostanes as an indicator and risk factor for coronary heart disease. Free Radic Biol Med 50(5): 559-566.
- Milne GL, Dai Q, Roberts II LJ (2015) The isoprostanes 25 years later. Biochimica et Biophysica Acta (BBA)-Molecular and Cell Biology of Lipids 1851(4): 433-445.
- Frijhoff J, Winyard PG, Zarkovic N, Davies SS, Stocker R (2015) Clinical relevance of biomarkers of oxidative stress. Antioxidants & redox signaling 23(14): 1144-1170.
- Ayala A, Muñoz MF, Argüelles S (2014) Lipid peroxidation: production, metabolism, and signaling mechanisms of malondialdehyde and 4-hydroxy-2-nonenal. Oxidative medicine and cellular longevity 2014: 360438.
- M Giera, H Lingeman, WMA Niessen (2012) Recent advancements in the LC- and GC-based analysis of malondialdehyde (MDA): a brief overview. Chromatographia 75(9-10): 433-440.
- Ansarin K, Khoubnasabjafari M, Jouyban A (2015) Reliability of malondialdehyde as a biomarker of oxidative stress in psychological disorders 5(3): 123-127.
- Davies MJ (2016) Protein oxidation and peroxidation. Biochemical Journal 473(7): 805-825.
- Meredith S, Parekh G, Towler J, Schouten J, Davis P (2014) Mapping nitro-tyrosine modifications in fibrinogen by mass spectrometry as a biomarker for inflammatory disease. Free Radical Biology and Medicine 75: S50.
- Jayakumari NR, Reghuvaran AC, Rajendran RS, Pillai VV, Karunakaran J (2014) Are nitric oxide-mediated protein modifications of functional significance in diabetic heart? ye'S,-NO’, wh ‘Y-Not? Nitric Oxide 43: 35-44.
- E Verhoye, MR Langlois, I Asklepios (2009) Circulating oxidized low-density lipoprotein: a biomarker of atherosclerosis and cardiovascular risk?” Clinical Chemistry and Laboratory Medicine 47(2): 128-137.
- P Holvoet, D De Keyzer, DR Jacobs Jr (2008) Oxidized LDL and the metabolic syndrome. Future Lipidology 3(6): 637-649.
- Trpkovic A, Resanovic I, Stanimirovic J, Radak D, Mousa SA (2015) Oxidized low-density lipoprotein as a biomarker of cardiovascular diseases. Crit Rev Clin Lab Sci 52(2): 70-85.
- GA Figtree, G Keyvan Karimi, CC Liu, HH Rasmussen (2012) Oxidative regulation of the Na(þ)-K(þ) pump in the cardiovascular system. Free Radical Biology and Medicine 53(12): 2263-2268.
- Chen CA, Wang TY, Varadharaj S, Reyes LA, Hemann C (2010) S-glutathionylation uncouples eNOS and regulates its cellular and vascular function. Nature 468(7327): 1115-1118.
- JR Burgoyne, H Mongue Din, P Eaton, AM Shah (2012) Redox signaling in cardiac physiology and pathology. Circulation Research 111(8): 1091-1106.
- Harrison D, Griendling KK, Landmesser U (2003) Role of Oxidative Stress in Atherosclerosis. regulation of vascular 91(3A): 7A-11A.
- Vogiatzi G, Tousoulis D, Stefanadis C (2009) The role of oxidative stress in atherosclerosis. Hellenic J cardiol 50(5): 402-409.
- Gielis JF, Lin JY, Wingler K (2011) Pathogenetic role of eNOS uncoupling in cardiopulmonary disorders. Free Radic Biol Med 50(7): 765-776.
- Dhawan V (2014) Reactive oxygen and nitrogen species: general considerations. In Dhawan V (Eds.), Studies on respiratory disorders. Humana Press, New York, NY pp. 27-47.
- Nishino T, Okamoto K, Eger BT (2008) Mammalian xanthine oxidoreductase̶ mechanism of transition from xanthine dehydrogenase to xanthine oxidase. FEBS J 275(13): 3278-3328.
- S Kojima, T Sakamoto, M Ishihara (2005) Coronary syndrome study, prognostic usefulness of serum uric acid after acute myocardial infarction (the Japanese Acute Coronary Syndrome Study). The American Journal of Cardiology 96(4): 489-495.
- Cantu Medellin, EE Kelley (2013) Xanthine oxidoreductase-catalyzed reactive species generation: Oxidative Medicine and Cellular Longevity process in critical need of reevaluation. Redox Biology 1(1): 353-358.
- LJ Ignarro, C Napoli (2005) Novel features on nitric oxide, endothelial nitric oxide synthase and atherosclerosis. Curr. Atheroscler R 5(1): 17-23.
- Napoli C, Nigris F De, Williams Ignarro S, Pignalosa O (2007) Nitric oxide and atherosclerosis : An update Nitric oxide and atherosclerosis. 15(4): 265-279.
- Senthilmohan R, Kettle AJ (2006) Bromination and chlorination reaction of myeloperoxidase at physical concentrations of bromide and chloride. Arch Biochem Biophys 445(2): 235-244.
- Osawa T (2018) Development and application of oxidative stress biomarkers. Biosci Biotechnol Biochem 82(4):564-572.
- SJ Nicholls, SL Hazen (2005) Myeloperoxidase and cardiovascular disease, Arteriosclerosis, Thrombosis, and Vascular Biology pp. 1102-1111.
- H Scharnagl, ME Kleber, B Genser (2014) Association of myeloperoxidase with total and cardiovascular mortality in individuals undergoing coronary angiography—the LURIC study. International Journal of Cardiology 174(1): 96-105.
- Koeth RA, Haselden V, Tang WH (2013) Myeloperoxidase in cardiovascular disease. Adv Clin Chem 62: 1-32.
- Stocker R, Huang A, Jeranian E (2004) Hypochlorous acid impairs endothelium-derived nitric oxide bioactivity through a superoxide-dependent mechanism. Arterioscl Thromb Vasc Biol 24(11): 2028-2033.
- Smith JD (2010) Dysfunctional HDL as a diagnostic and therapeutic target. Arterioscler Thromb Vasc Biol 30(2): 151-155.
- Shao B, Oda MN, Oram JF (2010) Myeloperoxidase: an oxidative pathway for generating dysfunctional highdensity lipoprotein. Chem Res Toxicol 23(3): 447-454.
- Fisher EA, Feig JE, Hewing B (2012) High-density lipoprotein function, dysfunction, and reverse cholesterol transport. Arterioscler Thromb Vasc Biol 32(12): 2813-2820.
- Nicholls SJ, Hazen SL (2009) Myeloperoxidase, modified lipoproteins, and atherogenesis. J Lipid Res 50(Suppl): S346-S351.
- Undurti A, Huang Y, Lupica JA (2009) Modification of high density lipoprotein by myeloperoxidase generates a pro-inflammatory particle. J Biol Chem 284(45): 30825-30835.
- Teng N, Maghzal GJ, Talib J, Rashid I, Lau AK (2017) The roles of myeloperoxidase in coronary artery disease and its potential implication in plaque rupture. Redox Report 22(2): 51-73.
- Jacob R (2018) Causal Role of Oxidants and Antioxidants in Atherosclerosis. Indian J. Cardiovasc. Dis. Women-WINCARS 3(1): 004-005.
- Mitra S, Deshmukh A, Sachdeva R, Lu J, Mehta JL (2011) Oxidized low-density lipoprotein and atherosclerosis implications in antioxidant therapy. Am J Med Sci 342(2): 135-142.
- Moradi F, Sewell RD, Lorigooini, Z, Rafieia Kopaei M (2018) Immunosuppression-lipid metabolism interplay and medicinal plants in atherosclerosis: A review Curr Pharm Des 24(24): 2789-2793.
- Kirichenko TV, Sobenin IA, Nikolic D, Rizzo M, Orekhov AN (2016) Anti-cytokine therapy for prevention of atherosclerosis. Phytomedicine 23(11): 1198-1210.
- Nikoforov NG, Gratchev AN, Sobenin IA, Orekhov AN, Kzhyhskowska YG (2013) Interaction of native and modified low density lipoprotein with intimal cells in atherosclerotic lesion. Patol Fiziol Eksp Ter 1: 109-117.
- Kishimoto Y, Yoshida H, Kondo K (2016) Potential anti-atherosclerotic properties of astaxanthin. Mar Drugs 14(2): 35.
- Libby P (2012) Inflammation in atherosclerosis. Arter. Thromb Vasc Biol 420: 868-874.
- Ouweneel AB, Van Eck M (2016) Lipoproteins as modulators of atherothrombosis: From endothelial function to primary and secondary coagulation. Vascul Pharmacol 82: 1-10.
- Salvayre R, Negre Salvayre A, Camaré C (2016) Oxidative theory of atherosclerosis and antioxidants. Biochimie 125: 281-296.
- Malek mohammad K, Sewell RDE (2019) Antioxidants and Atherosclerosis : Mechanistic Aspects 9(8): 301.